-
矿质元素的生物地球化学循环直接影响生态系统的生产力和稳定性。磷(P)作为碳、氮之外陆地生态系统最关键的营养元素,在植物体内的分配和代谢决定了植物的生长过程及生产力水平[1]。低磷环境在生态系统内普遍存在,土壤总磷库80%以上的磷不可移动[2],植物难以吸收利用。同时,土壤有效磷(Pi)易被铝离子、铁离子、钙离子吸附固定,与氢氧化合物构成螯合物[3−5],因此有效磷浓度随土壤发育而下降,导致世界范围内有效磷浓度低于10 µmol·L−1的土壤广泛存在[6]。
为满足农林业生产需求,集约施用磷肥成为生产经营的主要措施。然而,一般磷肥当季利用率仅为10%~25%,75%~90%的磷被转化固定成植物难以吸收的形态[7],导致磷肥利用率低下。同时,随着经济增长和人口剧增,过度开采和不合理的磷肥经营管理造成磷矿资源储备耗竭、水体磷素增加,对生态环境造成严重威胁[8−10]。近年来,关于土壤磷素循环研究的不断深入,揭示了植物进化形成的一系列复杂适应性策略[11−12],并发现土壤功能微生物对土壤磷库活化和植物营养健康有重要影响[13−14]。丛枝菌根真菌(arbuscular mycorrhizal fungi,AMF)和溶磷细菌(phosphate-solubilizing bacteria,PSB)在自然界土壤中普遍存在。研究发现,丛枝菌根真菌和溶磷细菌可以溶解难溶性无机磷、矿化有机磷,两者直接参与土壤磷素活化与植物磷素获取过程,与土壤、植物之间联系密切[15−16],在维持土壤磷养分有效性和生态系统功能中发挥重要作用[16−18]。鉴于此,为开发可持续土壤磷素高效利用途径,围绕植物-丛枝菌根真菌-溶磷细菌共生关系,详述了丛枝菌根真菌与溶磷细菌在植物磷养分吸收中的作用,强调了植物-丛枝菌根真菌-溶磷细菌互作增效对土壤磷素调动的途径和机制。
-
维管植物在长期进化过程中形成了多样化的根功能属性以提高对土壤磷资源的获取,维持体内磷稳态,包括根形态和根构型属性的响应[11−12, 19−20]、根分泌物属性的调节[3, 12]、磷素分配与再利用的调控[21−23]以及与菌根形成紧密的共生关系[24]。其中,光合碳投入是植物根系功能属性变化以促进高效获取地下资源的重要驱动力[25]。低磷条件下,植物光合产物优先向根系分配,部分作用于根系生长及其生理活动,促进根冠比增加[20, 26−27],改变根系特征性状,表现为根系比根长与侧根数量的增加[19, 28]、根系生长角度的调整[11, 19]及根毛长度与密度的改变[11, 29],从而提高根系与土壤界面的接触面积。拟南芥Arabidopsis thaliana在低磷水平下通过抑制主根生长,增加根系分支数量,促进侧根伸长生长,形成在土壤浅层分布、高度分支的根系结构[19]。在4种不同根系表型的菜豆Phaseolus vulgaris中根毛与根系生长角度协同影响植物的磷素吸收,低磷胁迫下根系表现为长且密的根毛形态与浅根型结构,可显著促进植株生物量的积累与磷素的吸收[29]。WEN等[28]对16种不同作物分析发现:根系直径与物种的磷养分获取策略关系密切,低磷环境中根系直径较细的物种通过促进根系分支、增加根系比根长和一级根系长度获取磷素,而粗根物种主要依靠丛枝菌根真菌的高定殖率来弥补低磷环境下根系形态表现的缺陷。因此,不同物种、基因型以及根系功能性状类型对磷胁迫的响应表现出不同的可塑性。
植物光合产物的另一流向则是以根系分泌物的形式释放到根际环境中,包括高分子量化合物酸性磷酸酶(APase)和低分子量化合物酚类、羧酸盐以及糖类等多种物质[12, 30−35]。WANG等[30]对拟南芥的研究表明:磷饥饿诱导根系分泌的APases可以释放到根际环境或滞留在根表面,通过水解有机磷,提高有效磷浓度。JUSZCZUK等[31]研究了长期磷酸盐饥饿对豆科Leguminosae植物根系酚类物质分泌量的影响,结果表明:缺磷植株根系酚类物质的分泌量是对照植株根系的5倍。HU等[32]也发现:儿茶酚、原儿茶酸、咖啡酸等酚类物质能够影响土壤磷有效性。与有机磷的矿化不同,根系释放的酚类物质主要通过与土壤中含磷矿物(如闭蓄态磷,O-P)结合,促进磷的解吸[35]。根系低分子量羧酸盐的分泌是植物磷吸收的重要策略,羧酸盐通过络合金属阳离子,并与磷竞争吸附位点的方式,从磷酸铁盐(Fe-P)、磷酸铝盐(Al-P)、闭蓄态磷素(O-P)等难溶性磷酸盐中释放有效磷[35]。ALMEIDA等[34]对3种禾本科Gramineae植物进行研究发现:磷饥饿均会诱导根系柠檬酸盐、异柠檬酸盐和苹果酸盐分泌的增加。TOUHAMI等[12]在禾本科和豆科植物的分泌物中则鉴定到更多种类,包括柠檬酸盐、苹果酸盐、丙二酸盐、乙酸盐、丙酮酸盐、乳酸盐、琥珀酸盐、富马酸盐和莽草酸盐。伴随着羧酸阴离子分泌的同时,根系也会释放氢离子(H+)对根际进行酸化[3],与羧酸阴离子协同作用于土壤难溶性磷酸盐[35],最终促进植物对磷素的吸收利用。此外,低磷导致植物体内APase、RNA酶(RNase)、磷酸烯醇式丙酮酸羧化酶(PEPC)、β-葡萄糖苷酶、ADPG焦磷酸化酶(AGPase)等内源酶促反应加剧[36−39],改变植物磷养分和碳同化物质的“库—源—流”格局[20, 22, 39−40],调控胁迫条件下根系系统发育[41]。根系主动或被动释放物质进入根际改变了根际土壤的物理、化学和生物学性质,使根际成为植物-微生物、微生物-微生物互作最活跃的热点区域[42],但根系分泌物的种类、分泌量与物种、基因型及植物的营养状况密切相关[34]。
-
菌根是自然界最重要的生物互作体系之一,丛枝菌根真菌占整个菌根群体的72%以上[43]。目前已经报道发现的丛枝菌根真菌接近300种[44],其中球囊霉科Glomeraceae为优势丛枝菌根真菌[45]。丛枝菌根真菌通过增加根圈范围[46]、表达高亲和力磷转运蛋白[47]、提高土壤磷酸酶活性[48]、分泌有机酸和糖类物质[49−51]等多种方式直接或间接作用于土壤特征及微生物类群,与植物养分吸收利用关系密切。
从资源经济角度看,宿主植物为共生关系的主导者,向丛枝菌根真菌提供满足生存、生长的碳水化合物,作为交换,丛枝菌根真菌向寄主植物提供矿质养分(如磷)[14],其资源交换强度随各自资源分配状态而调整[52],从而实现共生关系的双向控制。同时,丛枝菌根真菌、宿主植物的遗传背景以及土壤磷有效性影响植物-丛枝菌根真菌相互作用的成本与收益[53−56]。
-
菌根植物可以通过2种途径从土壤中获取磷素,即通过根表皮细胞和根毛进行吸收的直接途径以及通过丛枝菌根真菌菌丝进行吸收的菌丝途径,同时养分吸收的内部分工更加“社会化”[55−58]。由于植物体内外磷酸盐浓度差巨大,直接途径的养分吸收速度慢、碳资源投入大[55−59],而丛枝菌根真菌菌丝体存在高亲和力磷酸盐转运系统,并具有诱导植物体内产生高亲和力磷转运蛋白的能力[47, 55−56],是磷胁迫条件下植物磷素供应的主要途径[56, 60−61]。因此,菌丝途径的低碳成本以及吸收、转运的高效率使其成为植物获取和利用磷素的优势途径。
菌丝途径中,丛枝菌根真菌通过纤细的菌丝和广泛的分支网络改善宿主植物磷养分状态。外生菌丝在土壤中广泛生长,可延伸到离根表11.7 cm处,进入比根毛更小的土壤孔隙,增加吸收面积[46]。在扩大吸收范围的同时,丛枝菌根真菌菌丝体向菌丝际环境分泌多种有机物。TOLJANDER等[49]通过核磁共振光谱法分析了菌丝分泌液的组成物质,发现菌丝分泌物主要为甲酸、乙酸、葡萄糖和淀粉类似物;而LUTHFIANA等[62]在2种磷水平下收集了明根孢囊霉Rhizophagus clarus和异形根孢囊霉Rhizophagus irregularis的菌丝分泌液,发现除碳水化合物和大量有机酸外,还包括氨基酸、核酸、脂肪酸、植物激素等物质。外生菌丝的H+分泌在体外培养条件下被证实[63],当H+分泌时,菌丝际土壤发生酸化,菌丝际环境pH降低。此外,接种丛枝菌根真菌能够显著提高土壤磷酸酶活性[48, 64−65],与有机酸和其他菌丝分泌物共同作用影响菌根际、菌丝际过程,促进土壤有机磷矿化和难溶性磷酸盐(如Fe-P、Al-P、O-P)溶解,并表现出其他促进植物生长的作用[48−49, 65]。
菌根植物磷养分吸收的直接途径和菌丝途径相互作用[66],形成了菌根植物的综合磷吸收系统[55]。目前,采用磷的放射性同位素示踪方法[56, 60]解释植物在菌丝途径和直接途径间的平衡选择。磷养分供应充足条件下,丛枝菌根真菌的定殖和生长发育程度较低,直接途径在菌根植物的磷素获取途径中占据优势;磷养分匮乏条件下,丛枝菌根真菌的定殖和生长发育被促进,菌根植物偏好于通过菌丝途径从土壤中获取磷素,但菌丝途径的磷素贡献受到丛枝菌根真菌发育程度、土壤有效磷条件以及物种差异性等因素的影响[55−57]。SAWERS等[15]发现:根外菌丝长度与植物磷摄取量相关,并影响菌丝途径的磷素贡献;ZHANG等[55]的研究发现:土壤无机磷供给水平显著影响菌丝途径对植物磷素吸收的贡献率,而地上部磷需求可能决定了植物对2种吸收途径的权衡选择。此外,也有研究表明:植物种类、基因型和丛枝菌根真菌种类的差异也会影响菌丝途径的磷素贡献率[56−57, 67],导致不同丛枝菌根真菌-植物组合在植物磷吸收、生长方面的响应具有功能上的差异。
-
菌丝途径的磷养分吸收是以公平公正、互惠互利为原则的“贸易交换”过程[25, 68]。丛枝菌根真菌在宿主皮层细胞形成丛枝结构(丛枝周膜-真菌细胞壁-真菌细胞膜)的养分交换场所[57],外生菌丝则以H+-ATP酶为驱动力在土壤界面吸收磷酸盐,经体内转化为多聚磷酸盐后,通过细胞质流动运输至共生界面并释放[24, 57, 69]。作为交换,宿主植物以糖、脂肪酸等形式向真菌提供植株4%~20%的净光合产量[54, 70]。
高共生质量的丛枝菌根真菌-植物共生关系会发生频繁的养分交换行为。丛枝菌根真菌与宿主植物的资源交换由宿主主导,植物通过选择性分配碳资源,主动选择合作伙伴,并以减少根系碳分配的方式对表现不佳的丛枝菌根真菌进行制裁[71−72],而丛枝菌根真菌也会选择向提供更多碳水化合物的根系增加养分转移,进而实现稳定的合作关系[52]。
植物-丛枝菌根真菌共生关系的形成是共生体间养分交流的基础,在菌根植物系统性磷响应中,植物信号的释放影响植物-丛枝菌根真菌的合作关系,调控丛枝菌根真菌的发育。研究表明:独脚金内酯在磷缺乏下受到显著诱导,调控地上部生长发育过程的同时,作为根际信号,刺激丛枝菌根真菌的菌丝分支[73−75]。SHI等[76]发现:植物-丛枝菌根真菌共生关系的形成需要植物磷饥饿响应转录因子(PHRs)的参与,PHRs通过P1BS元件介导丛枝菌根真菌共生相关基因的表达,进而影响植物-丛枝菌根真菌的共生质量。此外,miR399在菌根植物叶片的表达增加,也被提出作为植物调节丛枝菌根真菌共生的信号分子,但高磷水平下过表达miR399无法恢复丛枝菌根真菌定殖[77],可能还涉及其他机制[57]。
-
溶磷细菌作为土壤微生物的重要群体,约占土壤细菌总体的1%~50%[17],多属于芽孢杆菌属Bacillus、假单胞菌属Pseudomonas和欧文氏菌属Erwinia,在土壤磷素的生物循环中发挥重要作用[13]。多数研究表明:溶磷细菌对磷酸铁盐(Fe-P)、磷酸铝盐(Al-P)或磷酸钙盐(Ca-P)和磷酸铅盐(Pb-P)等难溶性磷酸盐[78−81]具有良好的活化作用。由于其分布状态与群落结构存在显著的区域特异性、时空变异性和根际效应性[18, 82−85],不同类群溶磷细菌的溶磷效能、种群分布及其在土壤磷素活化过程中的微生物互作机制可能因环境、宿主变化产生显著差异[85−89]。
-
酸解是难溶性磷酸盐(包括Fe-P、Al-P、Ca-P、Pb-P、O-P等)在土壤中溶解的主要方式。类似于植物和丛枝菌根真菌,细菌也能够分泌释放低分子量有机酸或伴随呼吸、铵根离子(NH4 +)同化释放H+,影响土壤pH[90−93],而分泌物的种类、分泌量可能受到菌株类型、基因型的影响。SONG等[94]发现:洋葱伯克霍尔德菌株Burkholderia cepacia DA23释放的有机酸主要为葡萄糖酸;而吕俊等[95]发现:在马尾松Pinus massoniana根际土中分离得到的洋葱伯克霍尔德菌株则大量分泌柠檬酸、丙二酸等有机酸,表明细菌外分泌性有机酸种类存在差异,但有机酸的解离和H+的释放最终均会改变土壤磷素的有效性。
土壤有机磷的矿化与溶磷细菌外分泌性植酸酶、磷酸酶活性密切相关[96−99],大约30%~40%的可培养土壤微生物产生植酸酶并利用植酸(如酵母菌将植酸作为唯一的利用磷源)[97−98]。不可培养的微生物中虽然也存在大量植酸酶,但受限于技术手段,目前研究较少[24]。溶磷细菌普遍具有分泌磷酸酶矿化土壤有机磷的能力。HNAMTE等[99]对休耕和耕种2种土壤分离鉴定得到的44株溶磷细菌,庄馥璐等[89]在苹果Malus pumila根际土壤中分离纯化得到的10株溶磷细菌,均能产生磷酸酶,但不同菌株生产磷酸酶能力呈现差异。此外,LIU等 [100]在水稻Oryza sativa根际土壤中分离得到的3种巨大芽孢杆菌Bacillus megaterium均能合成铁载体,螯合Fe3+,表现出解磷能力。因此,溶磷细菌在土壤磷素资源的活化过程中具有相当贡献,而不同菌株种类和基因型差异可能导致溶磷细菌的解磷机制存在差异。
-
溶磷细菌作为土壤与植物根系间磷养分转化和转运的调节因子,受土壤环境因素影响的同时,与植物生长和生理代谢之间具有显著交互作用。包括植物根系在内的植物组织器官为土壤微生物提供能量,刺激其生长和代谢活动[101],释放碳水化合物、有机酸以及酶等化合物,为土壤微生物群体提供碳源[102]。相反,溶磷细菌通过合成植物激素[99−100, 103],刺激根系生长,增加根系与磷素的接触面积以及细菌与植物根际的接触几率[103−105]。KUDOYAROVA等[103]研究表明:溶磷菌株Paenibacillus illinoisensis IB 1087 和 Pseudomonas extremaustralis IB-Ki-13-1A可合成生长素(IAA),刺激根系生长;LIU等[100]发现:溶磷细菌Bacillus megaterium DD-2、Bacillus aryabhattai DD-3和Bacillus subtilis DD-4 均能产生IAA与赤霉素(GA),对植物的根系生长产生积极作用。溶磷细菌合成植物激素刺激根系生长的同时也会刺激根系分泌物的产生,从而为细菌的生长、生存提供更多碳源[104]。
接种溶磷细菌可显著改善植株光合作用,增加碳固定。PANHWAR等[106]发现:水稻接种Bacillus sp.可显著促进水稻光合作用;谯天敏等[107]在核桃Juglans sigllata上接种温哥华假单胞菌Pseudomonas vancouverensis PAN4,发现植株净光合速率和叶绿素含量均会增加,增幅随接种浓度增高而增大。此外,溶磷细菌能够抑制植株病原菌生长,降低病害发生。MENDES等[108]在甘蔗Saccharum officinarum中分离得到的洋葱伯克霍尔德菌株可以产生抗真菌的代谢物。ETMINANI等[109]发现:防御假单胞菌Pseudomonas protegens Pb78对植物病原菌Pseudomonas syringae pv. syringae Pss20 和 Pseudomonas tolaasii Pt18具有强烈的抑制作用。然而,植物与根际微生物的互作过程可能影响根部扩散屏障的形成,作用于根内生细菌群落和根际微生物群落组成[110],从而影响植物中矿质养分的平衡[111]以及胁迫的耐受性[83]。
-
虽然溶磷细菌和丛枝菌根真菌协调植物养分获取的过程存在一定差异,但溶磷细菌和丛枝菌根真菌在调控植物对土壤磷素的吸收和利用过程中并不孤立[17],存在积极的相互作用。丛枝菌根真菌缺少有机磷矿化相关基因,依赖溶磷细菌从有机复合物中释放磷素[112−113],而溶磷细菌的种类及丰度影响土壤有机磷库的矿化进程,可能决定了丛枝菌根真菌对土壤有机磷库的磷素获取能力[24, 113−114]。与根际微生物群落不同,丛枝菌根真菌可能具有高效溶磷菌株筛选的潜力,菌丝际菌群更具独特性、功能性[51]。NUCCIO等[115]通过根室和菌丝室分隔的双室培养系统发现:厚壁菌门Firmicutes类群对何氏球囊霉Glomus hoi有积极响应,而放线菌门Actinobacteria和丛毛单胞菌科Comamonadaceae类群对何氏球囊霉呈消极反应;ANDRADE等[116]对球囊霉属Glomus丛枝菌根真菌的菌丝际菌群鉴定发现:菌丝际菌群以芽孢杆菌属和节杆菌属Arthrobacter为主,根际以假单胞菌属为主,且不同丛枝菌根真菌处理菌丝际群落的多样性和丰度也存在差异;而WANG等[117]发现:在异形根孢囊霉Rhizophagus irregularis菌丝定殖的产碱假单胞菌Pseudomonas alcaligenes能够表现出溶磷功能,可能反映了丛枝菌根真菌菌丝对土壤微生物类群招募的选择性和对资源获取的需求性。
丛枝菌根真菌为细菌的移动提供物理结构和生存资源。丛枝菌根真菌外生菌丝在土壤中形成广泛的菌丝网络,溶磷细菌可以沿着菌丝表面水膜游动[118]到达根系表面和不同土壤空间,进而改变根际细菌群落[119]。然而由于土壤空间资源有效性时空斑块的存在,细菌群落的建立往往受到资源限制。丛枝菌根真菌通过外生菌丝体分泌植物光合产物,吸引和富集有益微生物[117]。ZHANG等[120]发现:丛枝菌根真菌可以分泌果糖吸引溶磷细菌富集,刺激细菌生长。JIANG等[118]也发现:菌丝分泌物刺激溶磷细菌数目的增加,但也有研究[121]认为:低磷条件下,溶磷细菌活性不受丛枝菌根真菌分泌物的刺激,同时存在磷养分竞争关系。
-
丛枝菌根真菌与溶磷细菌间的协同作用介导植物的生长表现[122−123]。在南方红豆杉Taxus chinensis var. mairei根系同时接种草木樨中华根瘤菌Sinorhizobium meliloti CHW10B与缩球囊霉Glomus constrictum可以改变根际微生物组的群落结构及丰度,促进南方红豆杉的生长[124];在玉米Zea mays根系接种链霉菌Streptomyces sp. W94和异形根孢囊霉时,异形根孢囊霉根外菌丝体的发育被促进,从而促进植株的根系生长和磷的吸收[125];同时,也有研究表明:在红三叶草Trifolium pratense 根系同时接种摩西斗管囊霉Funneliformis mosseae和假单胞菌Pseudomonas sp.时,两者的协同作用不仅对红三叶草的生长和养分吸收无积极影响,而且不利于丛枝菌根真菌侵染植物根系[126]。因此,丛枝菌根真菌与溶磷细菌间的协同作用对植物生长表现的影响具有高度的物种特异性,其中菌根的形成及其作用的发挥可能是影响植物生长表现的主要因素之一。
菌根辅助菌即对丛枝菌根真菌孢子萌发或菌丝生长发挥积极作用的菌丝际细菌[51, 127],主要包括假单胞菌属、芽孢杆菌属、类芽孢杆菌属 Paenibacillus、红球菌属Rhodococcus和链霉菌属Streptomyces[123, 125, 127−130],能够通过促进丛枝菌根真菌的定殖与发育影响植物性能表现,帮助丛枝菌根真菌与植物建立共生关系并将根际转变为菌根际。丛枝菌根真菌定殖过程中菌根辅助菌产生的IAA及其诱导的基因表达有助于菌根定殖期间的表型变化,进而促进定殖[131],而丛枝菌根真菌的成功定殖也会诱导植物激素水平[132]和根系分泌物化学成分[128]的改变,从而引起根系形态、结构的改变,同时在分泌物的数量和质量上影响根际微生物种群,使植物可以通过调节不同过程,灵活地做出响应。
-
植物-丛枝菌根真菌-溶磷细菌协同互作进行物质交流以适应养分胁迫环境的同时,也产生一系列信号交流,调控植物和微生物相关基因的表达[74, 133],进而可能推动微生物群落与植物的协同进化。植物、丛枝菌根真菌和溶磷细菌之间以丛枝菌根真菌为连接媒介,通过丛枝界面和菌丝际界面进行微调通信,菌丝际溶磷细菌影响植物-丛枝菌根真菌共生界面丛枝结构上碳-磷交换相关基因(MtPT4、MtHA1、STR)的表达水平[134],菌丝际界面溶磷细菌分泌磷酸酶和葡萄糖酸,矿化有机磷,溶解难溶性无机磷[121, 135],增加丛枝菌根真菌对有效磷的吸收和运输,进一步增强丛枝菌根真菌流向植物的磷通量以及植物向丛枝菌根真菌提供的碳通量。丛枝菌根真菌获得的碳,一部分用于丛枝菌根真菌自身生长发育,另一部分被丛枝菌根真菌外生菌丝转移到菌丝际环境招募溶磷细菌[117],同时形成特定的菌丝际菌群[113]。WANG等[136]在不同气候区实地采样,并利用受控盆栽实验证明了菌丝际微生物组虽然受到环境因素的显著影响,但存在以α变形菌纲Alphaproteobacteria、放线菌门Actinobacteria和γ变形菌纲Gammaproteobacteria为主的核心成员,且相对丰度与磷酸酶活性显著相关。因此,菌丝分泌物因其独特性可能极大地塑造了特定菌丝际细菌的定殖和功能,可能是驱动溶磷细菌与丛枝菌根真菌之间选择的有效信号。同时,丛枝菌根真菌通过提供不稳定碳源增加细菌的数量和活性,其中果糖可能是丛枝菌根真菌-溶磷细菌界面的一种信号分子[121, 135]。溶磷细菌可以通过响应丛枝菌根真菌分泌的果糖调节蛋白质分泌系统,刺激磷酸酶基因的表达以及磷酸酶释放到生长介质中的速率[136]。也有研究表明[137]:溶磷细菌在响应丛枝菌根真菌分泌物的同时也会刺激菌丝表面磷转运蛋白基因的表达,进而促进磷的转运。
细菌利用自身的信号转导系统响应菌丝分泌物信号。双元信号系统(TCS)是细菌普遍存在且非常保守的感知和响应外界刺激的调节组分,也是重要的代谢调节系统[138]。DUAN等[139]分析了水拉恩氏菌Rahnella aquatilis TCS中参与碳传感和营养传感的16个基因以及参与调控无机、有机磷活化的8个基因的表达水平,发现TCS在识别菌丝分泌物和菌丝际营养信号中起关键作用,有效地调控菌丝际磷素活性。此外,ZHANG等[140]发现:丛枝菌根真菌可能通过刺激参与细菌三羧酸(TCA)循环中柠檬酸合成酶基因(glt A)的表达,影响溶磷细菌三磷酸腺苷的生成水平,进而影响溶磷细菌的代谢。然而,由于基因的遗传冗余、共生体系培养方式及原位分析技术手段的限制,植物-丛枝菌根真菌-溶磷细菌共生体系间的信号交流在很大程度上仍然未知。
-
高效的磷养分循环是土壤、植物、微生物多界面互作的系统过程,磷养分胁迫下丛枝菌根真菌与溶磷细菌增强植物磷素获取能力的作用对植物生长和生产至关重要。根系作为植物吸收养分的主要器官,将地上和地下连为一体,控制着植物-土壤-微生物系统间的碳物质流动以在胁迫环境中招募丛枝菌根真菌、溶磷细菌定殖,并在土壤中形成以丛枝菌根真菌为纽带的“植物-根系-根际-丛枝菌根真菌-菌丝际-溶磷细菌”高度动态的根际生命共同体。菌丝际界面内丛枝菌根真菌与溶磷细菌的相互作用,提高了溶磷细菌矿化土壤有机磷和溶解难溶性无机磷的能力,促进了丛枝菌根真菌对土壤有效磷的吸收与转运,进而调控了植物对磷养分的获取、利用过程。现有研究探讨了受控条件下丛枝菌根真菌、溶磷细菌接种对植物生长发育表现的改善,多数研究局限于植物-丛枝菌根真菌、丛枝菌根真菌-溶磷细菌等单一性组合的作用结果,通常忽略了依赖于物种差异性、区域特异性以及时空变异性对植物-丛枝菌根真菌-溶磷细菌相互作用的功能产生的影响。
未来可以在以下几个方面进行深度研究:①丛枝菌根真菌在植物-丛枝菌根真菌-溶磷细菌互作体系中发挥关键作用,菌根属性是预测和理解生态系统磷养分循环的关键因素[141]。为深入了解菌根属性在植物-丛枝菌根真菌-溶磷细菌互作体系中的作用,破译互作体系作用机制,未来研究应在三者共生体系的基础上关注菌根形态、生理或物候特征与其功能属性的联系。②植物碳投入与收益间的平衡策略对深入理解植物-丛枝菌根真菌-溶磷细菌物质交换机制及其调控途径尤为重要,关注碳驱动下植物-根系-根际-丛枝菌根真菌-菌丝际-溶磷细菌体系的能量流动,分析、鉴定互作体系下植物、丛枝菌根真菌、溶磷细菌代谢物的组成及其潜在功能,对于丰富植物-根系-根际-土壤-微生物多界面互作增效,提高养分效率的相关理论基础具有重要意义。③在自然生态系统中大量的生物或非生物胁迫作用于植物-丛枝菌根真菌-溶磷细菌互作体系,进而影响其功能属性,使得田间条件下植物-丛枝菌根真菌-溶磷细菌互作增效作用机制持续成为一个重大的挑战。未来可深化土壤环境因素、土壤微生物竞争关系、生物菌剂施用对土壤微生物群落构建及其功能组装的影响研究,深入探讨人工合成菌群互作机制等途径,系统剖析可持续农林业生产的根际工程。
Mechanisms of plant P acquisition coordinated by arbuscular mycorrhizal fungi and phosphate-solubilizing bacteria
-
摘要: 磷素是生物生长发育的重要元素,溶磷细菌和丛枝菌根真菌直接参与土壤磷素活化和植物获取磷素的过程,对生态系统磷养分周转与植物产量的形成具有重要意义。从植物获取和利用磷的策略、植物磷素吸收的丛枝菌根真菌协调途径、植物磷素吸收的溶磷细菌协调途径、植物-丛枝菌根真菌-溶磷细菌的协同作用4个方面,总结分析了植物-微生物协作促进磷养分高效吸收利用的作用机制。分析发现:植物的磷素获取过程需要高效的根系适应能力,通过调控根系形态性状、改变根系分泌物成分与分泌量,促进土壤磷素活化;同时,丛枝菌根真菌可通过与植物间的互利共生物质交换,推动根际与菌丝际的土壤生物活性与化学性质变化,促进植物获取磷素;此外,溶磷细菌与植物、丛枝菌根真菌在土壤界面形成积极互作关系,分泌多种有机酸、降低土壤pH值、提高磷活化相关酶活性改善土壤可利用磷水平。在此基础上,对植物-丛枝菌根真菌-溶磷细菌互作促进植物磷素吸收的研究前景进行了展望。未来应重点关注:菌根属性在互作体系中的作用;分析鉴定互作体系成员的代谢物组成及其潜在功能;探讨生物或非生物因素对土壤微生物群落构建及其功能组装的影响。参141Abstract: Phosphorus is an important element for biological growth and development. Phosphate-solubilizing bacteria and arbuscular mycorrhizal fungi are directly involved in the process of soil phosphorus activation and plant phosphorus acquisition, which is of great significance for the turnover of phosphorus nutrients in ecosystems and the formation of plant yield. In this paper, the mechanism of plant-microorganism collaboration in promoting the efficient absorption and utilization of phosphorus nutrients was summarized and analyzed from four aspects: the strategy of plant phosphorus acquisition and utilization, the coordination pathway of arbuscular mycorrhizal fungi for plant phosphorus absorption, the coordination pathway of phosphate-solubilizing bacteria for plant phosphorus absorption, and the synergy of plant-arbuscular mycorrhizal fungi-phosphate-solubilizing bacteria. It was found in the analysis that the phosphorus acquisition process of plants required efficient root adaptability, which promoted soil phosphorus activation by regulating root morphological traits and changing the composition and secretion of root exudates. Arbuscular mycorrhizal fungi could promote the changes of soil biological activity and chemical properties in rhizosphere and hyphosphere by exchanging mutually beneficial symbiotic substances with plants, and promote plants to obtain phosphorus. Phosphate-solubilizing bacteria had a positive interaction with plants and arbuscular mycorrhizal fungi at the soil interface, secreting a variety of organic acids, reducing soil pH, and increasing the activities of phosphorus activation-related enzymes to improve soil available phosphorus levels. On this basis, research prospect of plant-arbuscular mycorrhizal fungi-phosphate-solubilizing bacteria interaction to promote plant phosphorus uptake was prospected. Future research should focus on the following aspects: the role of mycorrhizal traits in the interaction system, analysis and identification of metabolite composition and potential functions of the member of the interaction system, and to explore the effects of biotic or abiotic factors on the construction and functional assembly of soil microbial community. [Ch, 141 ref.]
-
[1] MARSCHNER P. Marschner’s Mineral Nutrition of Higher Plants [M]. 3th ed. San Diego: Academic Press, 2012: 3 − 5. [2] XU Xiaoli, MAO Xiali, van ZWIETEN L, et al. Wetting-drying cycles during a rice-wheat crop rotation rapidly (im) mobilize recalcitrant soil phosphorus [J]. Journal of Soils and Sediments, 2020, 20(9): 3921 − 3930. [3] HINSINGER P. Bioavailability of soil inorganic P in the rhizosphere as affected by root-induced chemical changes: a review [J]. Plant and Soil, 2001, 237: 173 − 195. [4] MA Jie, MA Yunling, WEI Rongfei, et al. Phosphorus transport in different soil types and the contribution of control factors to phosphorus retardation [J/OL]. Chemosphere, 2021, 276: 130012[2022-11-10]. doi: 10.1016/j.chemosphere.2021.130012. [5] ZHOU Jia, ZHANG Yufu, WU Kaibin, et al. National estimates of environmental thresholds for upland soil phosphorus in China based on a meta-analysis [J/OL]. Science of the Total Environment, 2021, 780(9): 146677[2022-11-10]. doi: 10.1016/j.scitotenv.2021.146677. [6] BIELESKI R L. Phosphate pools, phosphate transport, and phosphate availability [J]. Annual Review of Plant Physiology, 2003, 24(1): 225 − 252. [7] SYERS J K, JOHNSTON A E, CURTIN D. Efficiency of Soil and Fertilizer Phosphorus Use: Reconciling Changing Concepts of Soil Phosphorus Chemistry with Agronomic Information [M]. Rome: Food and Agriculture Organization of The United Nations, 2008: 108. [8] VANCE C P, UHDE-STONE C, ALLAN D L. Phosphorus acquisition and use: critical adaptations by plants for securing a nonrenewable resource [J]. New Phytologist, 2003, 157(3): 423 − 447. [9] ELSER J, BENNETT E. Phosphorus cycle: a broken biogeochemical cycle [J]. Nature, 2011, 478(7367): 29 − 31. [10] SCHERER L, PFISTER S. Modelling spatially explicit impacts from phosphorus emissions in agriculture [J]. International Journal of Life Cycle Assessment, 2015, 20: 785 − 795. [11] LYNCH J P. Root phenotypes for improved nutrient capture: an underexploited opportunity for global agriculture [J]. New Phytologist, 2019, 223(2): 548 − 564. [12] TOUHAMI D, MCDOWELL R W, CONDRON L M. Role of organic anions and phosphatase enzymes in phosphorus acquisition in the rhizospheres of legumes and grasses grown in a low phosphorus pasture soil [J/OL]. Plants, 2020, 9(9): 1185[2022-11-10]. doi: 10.3390/plants9091185. [13] BAHADUR B, RAJAM M V, SAHIJRAM L, et al. Plant Biology and Biotechnology [M]. New Delhi: Springer, 2015: 307 − 333. [14] GENRE A, LANFRANCO L, PEROTTO S, et al. Unique and common traits in mycorrhizal symbioses [J]. Nature Reviews Microbiology, 2020, 18(11): 649 − 660. [15] SAWERS R J H, SVANE S F, QUAN C, et al. Phosphorus acquisition efficiency in arbuscular mycorrhizal maize is correlated with the abundance of root-external hyphae and the accumulation of transcripts encoding PHT1 phosphate transporters [J]. New Phytologist, 2017, 214(2): 632 − 643. [16] BI Qingfang, ZHENG Bangxiao, LIN Xianyong, et al. The microbial cycling of phosphorus on long-term fertilized soil: insights from phosphate oxygen isotope ratios [J]. Chemical Geology, 2018, 483: 56 − 64. [17] SHARMA S B, SAYYED R Z, TRIVEDI M H, et al. Phosphate solubilizing microbes: sustainable approach for managing phosphorus deficiency in agricultural soils [J/OL]. Springer Plus, 2013, 2: 587[2022-11-10]. doi:10.1186/2193-1801-2-587. [18] XING Yijing, SHI Wenhui, ZHU Ying, et al. Screening and activity assessing of phosphorus availability improving microorganisms associated with bamboo rhizosphere in subtropical China [J]. Environmental Microbiology, 2021, 23(10): 6074 − 6088. [19] WILLIAMSON L C, RIBRIOUX S P C P, FITTER A H, et al. Phosphate availability regulates root system architecture in Arabidopsis [J]. Plant Physiology, 2001, 126(2): 875 − 882. [20] 周建菲, 史文辉, 潘凯婷, 等. 低磷胁迫对毛竹幼苗生长和养分生理的影响[J]. 浙江农林大学学报, 2022, 39(5): 1010 − 1017. ZHOU Jianfei, SHI Wenhui, PAN Kaiting, et al. Effect of low phosphorus stress on growth and nutrient physiology of Phyllostachys edulis seedling [J]. Journal of Zhejiang A&F University, 2022, 39(5): 1010 − 1017. [21] LÓPEZ-ARREDONDO D L, LEYVA-GONZÁLEZ M A, GONZÁLEZ-MORALES S I, et al. Phosphate nutrition: improving low-phosphate tolerance in crops [J]. Annual Review of Plant Biology, 2014, 65: 95 − 123. [22] WANG Ruzhen, CRESSWELL T, JOHANSEN M P, et al. Re-allocation of nitrogen and phosphorus from roots drives regrowth of grasses and sedges after defoliation under deficit irrigation and nitrogen enrichment [J]. Journal of Ecology, 2021, 109(12): 4071 − 4080. [23] VENEKLAAS E J, LAMBERS H, BRAGG J, et al. Opportunities for improving phosphorus-use efficiency in crop plants [J]. New Phytologist, 2012, 195(2): 306 − 320. [24] TIAN Jiang, GE Fei, ZHANG Dayi, et al. Roles of phosphate solubilizing microorganisms from managing soil phosphorus deficiency to mediating biogeochemical P cycle [J/OL]. Biology, 2021, 10(2): 158[2022-11-10]. doi: 10.3390/biology10020158. [25] LYNCH J P, HO M D. Rhizoeconomics: carbon costs of phosphorus acquisition [J]. Plant and Soil, 2005, 269: 45 − 56. [26] HAMMELEHLE A, OBERSON A, LÜSCHER A, et al. Above- and belowground nitrogen distribution of a red clover-perennial ryegrass sward along a soil nutrient availability gradient established by organic and conventional cropping systems [J]. Plant and Soil, 2018, 425: 507 − 525. [27] 胡支舰, 庚金武, 陈健, 等. 氮沉降对低磷胁迫下毛竹实生幼苗生长及土壤化学特性的影响[J]. 浙江林业科技, 2021, 41(2): 17 − 22. HU Zhijian, GENG Jinwu, CHEN Jian, et al. Effect of nitrogen deposition on growth of Phyllostachys edulis seedlings and soil chemical properties under low phosphorus stress [J]. Journal of Zhejiang Forestry Science and Technology, 2021, 41(2): 17 − 22. [28] WEN Zhihui, LI Hongbo, SHEN Qi, et al. Tradeoffs among root morphology, exudation and mycorrhizal symbioses for phosphorus-acquisition strategies of 16 crop species [J]. New Phytologist, 2019, 223(2): 882 − 895. [29] MIGUEL M A, POSTMA J A, LYNCH J P. Phene synergism between root hair length and basal root growth angle for phosphorus acquisition [J]. Plant Physiology, 2015, 167(4): 1430 − 1439. [30] WANG Liangsheng, LIU Dong. Analyses of root-secreted acid phosphatase activity in Arabidopsis [J/OL]. Bio-Protocol, 2017, 7(7): e2202[2022-11-15]. doi: 10.21769/BioProtoc.2202. [31] JUSZCZUK I M, WIKTOROWSKA A, MALUSÁ E, et al. Changes in the concentration of phenolic compounds and exudation induced by phosphate deficiency in bean plants (Phaseolus vulgaris L.) [J]. Plant and Soil, 2004, 267(1): 41 − 49. [32] HU H, TANG C, RENGEL Z. Influence of phenolic acids on phosphorus mobilisation in acidic and calcareous soils [J]. Plant and Soil, 2005, 268: 173 − 180. [33] JONES D L, DENNIS P G, OWEN A G, et al. Organic acid behavior in soils-misconceptions and knowledge gaps [J]. Plant and Soil, 2003, 248(1): 31 − 41. [34] ALMEIDA D S, DELAI L B, SAWAYA A C H F, et al. Exudation of organic acid anions by tropical grasses in response to low phosphorus availability [J/OL]. Scientific Reports, 2020, 10(1): 16955[2022-11-15]. doi: 10.1038/s41598-020-73398-1. [35] CHAI Y N, SCHACHTMAN D P. Root exudates impact plant performance under abiotic stress [J]. Trends in Plant Science, 2022, 27(1): 80 − 91. [36] RICHARDSON A E. Regulating the phosphorus nutrition of plants: molecular biology meeting agronomic needs [J]. Plant and Soil, 2009, 322: 17 − 24. [37] PLAXTON W C, TRAN H T. Metabolic adaptations of phosphate-starved plants [J]. Plant Physiology, 2011, 156(3): 1006 − 1015. [38] DENG Minjun, HU Bin, XU Lei, et al. OsCYCP1;1, a PHO80 homologous protein, negatively regulates phosphate starvation signaling in the roots of rice (Oryza sativa L.) [J]. Plant Molecular Biology, 2014, 86(6): 655 − 669. [39] 王保明, 陈永忠, 王湘南, 等. 植物低磷胁迫响应及其调控机制[J]. 福建农林大学学报 (自然科学版), 2015, 44(6): 567 − 575. WANG Baoming, CHEN Yongzhong, WANG Xiangnan, et al. The response to low phosphorus stress and its regulation mechanism in plants [J]. Journal of Fujian Agriculture and Forestry University (Natural Science Edition), 2015, 44(6): 567 − 575. [40] XU Lei, ZHAO Hongyu, WAN Renjing, et al. Identification of vacuolar phosphate efflux transporters in land plants [J]. Nature Plants, 2019, 5(1): 84 − 94. [41] LIN Weiyi, HUANG Tengkuei, LEONG Shangjye, et al. Long-distance call from phosphate: systemic regulation of phosphate starvation responses [J]. Journal of Experimental Botany, 2014, 65(7): 1817 − 1827. [42] ZHANG Deshan, ZHANG Chaochun, TANG Xiaoyan, et al. Increased soil phosphorus availability induced by faba bean root exudation stimulates root growth and phosphorus uptake in neighbouring maize [J]. New Phytologist, 2016, 209(2): 823 − 831. [43] BRUNDRETT M C. Mycorrhizal associations and other means of nutrition of vascular plants: understanding the global diversity of host plants by resolving conflicting information and developing reliable means of diagnosis [J]. Plant and Soil, 2009, 320: 37 − 77. [44] 王幼珊, 张淑彬, 殷晓芳, 等. 中国大陆地区丛枝菌根真菌菌种资源的分离鉴定与形态学特征[J]. 微生物学通报, 2016, 43(10): 2154 − 2165. WANG Youshan, ZHANG Shubin, YIN Xiaofang, et al. Isolation and identification of arbuscular mycorrhizal fungi from mainland China [J]. Microbiology China, 2016, 43(10): 2154 − 2165. [45] DIAGNE N, NGOM M, DJIGHALY P I, et al. Roles of arbuscular mycorrhizal fungi on plant growth and performance: importance in biotic and abiotic stressed regulation [J/OL]. Diversity, 2020, 12(10): 370[2022-11-15]. doi: 10.3390/d12100370. [46] SMITH S E, SMITH F A. Roles of arbuscular mycorrhizas in plant nutrition and growth: new paradigms from cellular to ecosystem scales [J]. Annual Review of Plant Biology, 2011, 62: 227 − 250. [47] XIE Xian’an, LAI Wenzhen, CHE Xianrong, et al. A SPX domain-containing phosphate transporter from Rhizophagus irregularis handles phosphate homeostasis at symbiotic interface of arbuscular mycorrhizas [J]. New Phytologist, 2022, 234(2): 650 − 671. [48] MÓNICA I F D, GODEAS A M, SCERVINO J M. In vivo modulation of arbuscular mycorrhizal symbiosis and soil quality by fungal P solubilizers [J]. Microbial Ecology, 2020, 79(1): 21 − 29. [49] TOLJANDER J F, LINDAHL B D, PAUL L R, et al. Influence of arbuscular mycorrhizal mycelial exudates on soil bacterial growth and community structure [J]. FEMS Microbiology Ecology, 2007, 61(2): 295 − 304. [50] MACKAY J E, CAVAGNARO T R, MÜLLER S D S, et al. A key role for arbuscular mycorrhiza in plant acquisition of P from sewage sludge recycled to soil [J]. Soil Biology and Biochemistry, 2017, 115: 11 − 20. [51] ZHANG Lin, ZHOU Jiachao, GEORGE T S, et al. Arbuscular mycorrhizal fungi conducting the hyphosphere bacterial orchestra [J]. Trends in Plant Science, 2022, 27(4): 402 − 411. [52] KIERS E T, DUHAMEL M, BEESETTY Y, et al. Reciprocal rewards stabilize cooperation in the mycorrhizal symbiosis [J]. Science, 2011, 333(6044): 880 − 882. [53] CHEN Min, ARATO M, BORGHI L, et al. Beneficial services of arbuscular mycorrhizal fungi-from ecology to application [J/OL]. Frontiers in Plant Science, 2018, 9: 1270[2022-11-15]. doi: 10.3389/fpls.2018.01270. [54] BENNETT A E, GROTEN K. The costs and benefits of plant-arbuscular mycorrhizal fungal interactions [J]. Annual Review of Plant Biology, 2022, 73: 649 − 672. [55] ZHANG Lin, CHU Qun, ZHOU Jianwei, et al. Soil phosphorus availability determines the preference for direct or mycorrhizal phosphorus uptake pathway in maize [J/OL]. Geoderma, 2021, 403: 115261[2022-11-15]. doi: 10.1016/j.geoderma.2021.115261. [56] CHU Qun, ZHANG Lin, ZHOU Jianwei, et al. Soil plant-available phosphorus levels and maize genotypes determine the phosphorus acquisition efficiency and contribution of mycorrhizal pathway [J]. Plant and Soil, 2020, 449: 357 − 371. [57] FERROL N, AZCÓN-AGUILAR C, PÉREZ-TIENDA J. Review: arbuscular mycorrhizas as key players in sustainable plant phosphorus acquisition: an overview on the mechanisms involved [J]. Plant Science, 2019, 280: 441 − 447. [58] SMITH S E, JAKOBSEN I, GRØNLUND M, et al. Roles of arbuscular mycorrhizas in plant phosphorus nutrition: interactions between pathways of phosphorus uptake in arbuscular mycorrhizal roots have important implications for understanding and manipulating plant phosphorus acquisition [J]. Plant Physiology, 2011, 156(3): 1050 − 1057. [59] BERGMANN J, WEIGELT A, van der PLAS F, et al. The fungal collaboration gradient dominates the root economics space in plants [J/OL]. Science Advances, 2020, 6(27): eaba3756[2022-11-15]. doi: 10.1126/sciadv.aba3756. [60] SMITH S E, SMITH F A, JAKOBSEN I. Mycorrhizal fungi can dominate phosphate supply to plants irrespective of growth responses [J]. Plant Physiology, 2003, 133(1): 16 − 20. [61] SCHNEPF A, ROOSE T, SCHWEIGER P. Impact of growth and uptake patterns of arbuscular mycorrhizal fungi on plant phosphorus uptake-a modelling study [J]. Plant and Soil, 2008, 312: 85 − 99. [62] LUTHFIANA N, INAMURA N, TANTRIANI, et al. Metabolite profiling of the hyphal exudates of Rhizophagus clarus and Rhizophagus irregularis under phosphorus deficiency [J]. Mycorrhiza, 2021, 31(3): 403 − 412. [63] BHARADWAJ D P, ALSTRÖM S, LUNDQUIST P O. Interactions among Glomus irregulare, arbuscular mycorrhizal spore-associated bacteria, and plant pathogens under in vitro conditions [J]. Mycorrhiza, 2012, 22(6): 437 − 447. [64] KUCEY R M N. Phosphate-solubilizing bacteria and fungi in various cultivated and virgin alberta soils [J]. Canadian Journal of Soil Science, 1983, 63(4): 671 − 678. [65] 姚青. 植物对VA菌根的依赖性差异及菌根活化难溶性磷酸盐的机理研究 [D]. 北京: 中国农业大学, 2000. YAO Qing. Variation of Plants Myeorrhizal Dependency and Mobilization of Scareely-soluble Phosphate by VA Mycorrhizae [D]. Beijing: China Agricultural University, 2000. [66] NAGY R, DRISSNER D, AMRHEIN N, et al. Mycorrhizal phosphate uptake pathway in tomato is phosphorus-repressible and transcriptionally regulated [J]. New Phytologist, 2009, 181(4): 950 − 959. [67] 任爱天, 鲁为华, 杨洁晶, 等. 不同磷水平下AM真菌对紫花苜蓿生长和磷利用的影响[J]. 中国草地学报, 2014, 36(6): 72 − 78. REN Aitian, LU Weihua, YANG Jiejing, et al. Effects of arbuscular mycorrhizal fungi (AMF) on growth of alfalfa and phosphorus utilization under different P levels [J]. Chinese Journal of Grassland, 2014, 36(6): 72 − 78. [68] HONVAULT N, HOUBEN D, NOBILE C, et al. Tradeoffs among phosphorus-acquisition root traits of crop species for agroecological intensification [J]. Plant and Soil, 2021, 461: 137 − 150. [69] BÜNEMANN E K, OBERSON A, FROSSARD E, et al. Phosphorus in Action-biological Processes in Soil Phosphorus Cycling [M]. Berlin: Springer, 2011: 137. [70] JIANG Yina, WANG Wanxiao, XIE Qiujin, et al. Plants transfer lipids to sustain colonization by mutualistic mycorrhizal and parasitic fungi [J]. Science, 2017, 356(6343): 1172 − 1175. [71] FOSTER K R, KOKKO H. Cheating can stabilize cooperation in mutualisms [J]. Proceedings of the Royal Society B:Biological Sciences, 2006, 273(1598): 2233 − 2239. [72] AKÇAY E. Evolutionary Models of Mutualism [M]. New York: Oxford University Press, 2015: 57 − 76. [73] KOHLEN W, CHARNIKHOVA T, LIU Qing, et al. Strigolactones are transported through the xylem and play a key role in shoot architectural response to phosphate deficiency in nonarbuscular mycorrhizal host Arabidopsis [J]. Plant Physiologist, 2011, 155(2): 974 − 987. [74] OLDROYD G E, LEYSER O. A plant’s diet, surviving in a variable nutrient environment [J/OL]. Science, 2020, 368(6486): eaba0196[2022-11-15]. doi: 10.1126/science.aba0196. [75] AKIYAMA K, MATSUZAKI K, HAYASHI H. Plant sesquiterpenes induce hyphal branching in arbuscular mycorrhizal fungi [J]. Nature, 2005, 435: 824 − 827. [76] SHI Jincai, ZHAO Boyu, ZHENG Shuang, et al. A phosphate starvation response-centered network regulates mycorrhizal symbiosis [J]. Cell, 2021, 184(22): 5527 − 5540. [77] BRANSCHEID A, SIEH D, PANT B D, et al. Expression pattern suggests a role of MiR399 in the regulation of the cellular response to local Pi increase during arbuscular mycorrhizal symbiosis [J]. Molecular Plant-Microbe Interactions, 2010, 23(7): 915 − 926. [78] 赵小蓉, 林启美, 李保国. 溶磷菌对4种难溶性磷酸盐溶解能力的初步研究[J]. 微生物学报, 2002, 42(2): 236 − 241. ZHAO Xiaorong, LIN Qimei, LI Baoguo. The solubilization of four insoluble phosphates by some microorganisms [J]. Acta Microbiologica Sinica, 2002, 42(2): 236 − 241. [79] ILLMER P, SCHINNER F. Solubilization of inorganic calcium phosphates-solubilization mechanisms [J]. Soil Biology and Biochemistry, 1995, 27(3): 257 − 263. [80] 冯哲叶, 陈莎莎, 王文超, 等. 几株溶磷细菌的筛选和鉴定及其溶磷效果[J]. 南京农业大学学报, 2017, 40(5): 842 − 849. FENG Zheye, CHEN Shasha, WANG Wenchao, et al. Screening and identification of several phosphate-solubilizing bacteria and effect of their P-solubility [J]. Journal of Nanjing Agricultural University, 2017, 40(5): 842 − 849. [81] XU Jiacheng, HUANG Limin, CHEN Chengyu, et al. Effective lead immobilization by phosphate rock solubilization mediated by phosphate rock amendment and phosphate solubilizing bacteria [J/OL]. Chemosphere, 2019, 237: 124540[2022-11-15]. doi: 10.1016/j.chemosphere.2019.124540. [82] 池景良, 郝敏, 王志学, 等. 解磷微生物研究及应用进展[J]. 微生物学杂志, 2021, 41(1): 1 − 7. CHI Jingliang, HAO Min, WANG Zhixue, et al. Advances in research and application of phosphorus-solubilizing microorganism [J]. Journal of Microbiology, 2021, 41(1): 1 − 7. [83] BENNING J W, MOELLER D A. Microbes, mutualism, and range margins: testing the fitness consequences of soil microbial communities across and beyond a native plant’s range [J]. New Phytologist, 2021, 229(5): 2886 − 2900. [84] SAHU P K, SINGH D P, PRABHA R, et al. Connecting microbial capabilities with the soil and plant health: options for agricultural sustainability [J]. Ecological Indicators, 2019, 105: 601 − 612. [85] SHI Wenhui, XING Yijing, ZHU Ying, et al. Diverse responses of pqqC- and phoD-harbouring bacterial communities to variation in soil properties of moso bamboo forests [J]. Microbial Biotechnology, 2022, 15(7): 2097 − 2111. [86] BERNARD J, WALL C B, COSTANTINI M S, et al. Plant part and a steep environmental gradient predict plant microbial composition in a tropical watershed [J]. The ISME Journal, 2021, 15: 999 − 1009. [87] YAO Fei, YANG Shan, WANG Zhirui, et al. Microbial taxa distribution is associated with ecological trophic cascades along an elevation gradient [J/OL]. Frontiers in Microbiology, 2017, 8: 2071[2022-11-15]. doi: 10.3389/fmicb.2017.02071. [88] CHEN Yepei, REKHA P D, ARUN A B, et al. Phosphate solubilizing bacteria from subtropical soil and their tricalcium phosphate solubilizing abilities [J]. Applied Soil Ecology, 2006, 34(1): 33 − 41. [89] 庄馥璐, 柴小粉, 高蓓蓓, 等. 苹果根际解磷菌的分离筛选及解磷能力[J]. 中国农业大学学报, 2020, 25(7): 69 − 79. ZHUANG Fulu, CHAI Xiaofen, GAO Beibei, et al. Isolation and screening of phosphorus-solubilizing bacteria in apple rhizosphere [J]. Journal of China Agricultural University, 2020, 25(7): 69 − 79. [90] KIM K Y, MC DONALD G A, JORDAN D. Solubilization of hydroxyapatite by Enterobacter agglomerans and cloned Escherichia coli in culture medium [J]. Biology and Fertility of Soils, 1997, 24(4): 347 − 352. [91] PARK K H, LEE C Y, SON H J, et al. Mechanism of insoluble phosphate solubilization by Pseudomonas fluorescens RAF15 isolated from ginseng rhizosphere and its plant growth-promoting activities [J]. Letters in Applied Microbiology, 2009, 49: 222 − 228. [92] AWASTHI R, TEWARI R, NAYYAR H. Synergy between plants and P-solubilizing microbes in soils: effects on growth and physiology of crops [J]. International Research Journal of Microbiology, 2011, 2(12): 484 − 503. [93] 薛冬, 黄向东, 杨瑞先, 等. 牡丹根际溶磷放线菌的筛选及其溶磷特性[J]. 应用生态学报, 2018, 29(5): 1645 − 1652. XUE Dong, HUANG Xiangdong, YANG Ruixian, et al. Screening and phosphate-solubilizing characteristics of phosphate-solubilizing actinomycetes in rhizosphere of tree peony [J]. Chinese Journal of Applied Ecology, 2018, 29(5): 1645 − 1652. [94] SONG O R, LEE S J, LEE Y S, et al. Solubilization of insoluble inorganic phosphate by Burkholderia cepacia DA23 isolated from cultivated soil [J]. Brazilian Journal of Microbiology, 2008, 39(1): 151 − 156. [95] 吕俊, 于存. 1株高效溶磷伯克霍尔德菌的筛选鉴定及对马尾松幼苗的促生作用[J]. 应用生态学报, 2020, 31(9): 2923 − 2934. LÜ Jun, YU Cun. Screening and identification of an efficient phosphate-solubilizing Burkholderia sp. and its growth-promoting effect on Pinus massoniana seedling [J]. Chinese Journal of Applied Ecology, 2020, 31(9): 2923 − 2934. [96] LIDBURY I D E A, SCANLAN D J, MURPHY A R J, et al. A widely distributed phosphate-insensitive phosphatase presents a route for rapid organophosphorus remineralization in the biosphere [J/OL]. Proceedings of the National Academy of Sciences of the United States of America, 2022, 119(5): e2118122119[2022-11-15]. doi: 10.1073/pnas. 2118122119. [97] LIANG Xinjin, CSETENYI L, GADD G M. Lead bioprecipitation by yeasts utilizing organic phosphorus substrates [J]. Geomicrobiology Journal, 2016, 33(3/4): 294 − 307. [98] RODRÍGUEZ H, VIDAL R F. Phosphate solubilizing bacteria and their role in plant growth promotion [J]. Biotechnology Advances, 1999, 17(4/5): 319 − 339. [99] HNAMTE R, LALRUATSANGA H, LALFAKZUALA R. Isolation, identification and characterization of phosphate solubilizing bacteria from Jhum Field of Mizoram, India [J]. Geomicrobiology Journal, 2021, 38: 924 − 933. [100] LIU Zeping, ZHANG Xiaolong, LI Leibing, et al. Isolation and characterization of three plant growth-promoting rhizobacteria for growth enhancement of rice seedling [J]. Journal of Plant Growth Regulation, 2022, 41: 1382 − 1393. [101] 孙波, 廖红, 苏彦华, 等. 土壤-根系-微生物系统中影响氮磷利用的一些关键协同机制的研究进展[J]. 土壤, 2015, 47(2): 210 − 219. SUN Bo, LIAO Hong, SU Yanhua, et al. Advances in key coordinative mechanisms in soil-root-microbe systems to affect nitrogen and phosphorus utilization [J]. Soils, 2015, 47(2): 210 − 219. [102] TIAN Baoliang, PEI Yingchun, HUANG Wei, et al. Increasing flavonoid concentrations in root exudates enhance associations between arbuscular mycorrhizal fungi and an invasive plant [J]. The ISME Journal, 2021, 15(7): 1919 − 1930. [103] KUDOYAROVA G R, VYSOTSKAYA L B, ARKHIPOVA T N, et al. Effect of auxin producing and phosphate solubilizing bacteria on mobility of soil phosphorus, growth rate, and P acquisition by wheat plants [J/OL]. Acta Physiologiae Plantarum, 2017, 39(11): 253[2022-11-15]. doi: 10.1007/s11738-017-2556-9. [104] KUDOYAROVA G R, MELENTIEV A I, MARTYNENKO E V, et al. Cytokinin producing bacteria stimulate amino acid deposition by wheat roots [J]. Plant Physiology and Biochemistry, 2014, 83: 285 − 291. [105] HAYAT R, ALI S, AMARA U, et al. Soil beneficial bacteria and their role in plant growth promotion: a review [J]. Annals of Microbiology, 2010, 60(4): 579 − 589. [106] PANHWAR Q A, OTHMAN R, NAHER U A, et al. Effect of phosphate-solubilizing bacteria and oxalic acid on phosphate uptake from different P fractions and growth improvement of aerobic rice using 32P technique [J]. Australian Journal of Crop Science, 2013, 7(8): 1131 − 1140. [107] 谯天敏, 李姝江, 韩珊, 等. 温哥华假单胞菌菌株PAN4解磷能力及对核桃的促生作用[J]. 华南农业大学学报, 2015, 36(5): 117 − 124. QIAO Tianmin, LI Shujiang, HAN Shan, et al. The phosphate-solubilizing ability and growth promoting effects of Pseudomonas vancouverensis strain PAN4 on walnut [J]. Journal of South China Agricultural University, 2015, 36(5): 117 − 124. [108] MENDES R, PIZZIRANI-KLEINER A A, ARAÚJO W L, et al. Diversity of cultivated endophytic bacteria from sugarcane: genetic and biochemical characterization of Burkholderia cepacia complex isolates [J]. Applied and Environmental Microbiology, 2007, 73(22): 7259 − 7267. [109] ETMINANI F, BEHROUZ H. Isolation and identification of endophytic bacteria with plant growth promoting activity and biocontrol potential from wild pistachio trees [J]. The Plant Pathology Journal, 2018, 34(3): 208 − 217. [110] BHALLA K, QU Xianya, KRETSCHMER M, et al. The phosphate language of fungi [J]. Trends Microbiology, 2022, 30(4): 338 − 349. [111] SALAS-GONZÁLEZ I, REYT G, FLIS P, et al. Coordination between microbiota and root endodermis supports plant mineral nutrient homeostasis [J/OL]. Science, 2021, 371(6525): eabd0695[2022-11-15]. doi: 10.1126/science.abd0695. [112] SUN Xuepeng, CHEN Wenbo, IVANOV S, et al. Genome and evolution of the arbuscular mycorrhizal fungus Diversispora epigaea (formerly Glomus versiforme) and its bacterial endosymbionts [J]. New Phytologist, 2019, 221(3): 1556 − 1573. [113] EMMETT B D, LÉVESQUE-TREMBLAY V, HARRISON M J. Conserved and reproducible bacterial communities associate with extraradical hyphae of arbuscular mycorrhizal fungi [J]. The ISME Journal, 2021, 15(8): 2276 − 2288. [114] TISSERANT E, MALBREIL M, KUO A, et al. Genome of an arbuscular mycorrhizal fungus provides insight into the oldest plant symbiosis [J]. Proceedings of the National Academy of Sciences, 2013, 110(50): 20117 − 20122. [115] NUCCIO E E, HODGE A, PETT-RIDGE J, et al. An arbuscular mycorrhizal fungus significantly modifies the soil bacterial community and nitrogen cycling during litter decomposition [J]. Environmental Microbiology, 2013, 15(6): 1870 − 1881. [116] ANDRADE G, MIHARA K L, LINDERMAN R G, et al. Bacteria from rhizosphere and hyphosphere soils of different arbuscular-mycorrhizal fungi [J]. Plant and Soil, 1997, 192: 71 − 79. [117] WANG Fei, SHI Ning, JIANG Rongfeng, et al. In situ stable isotope probing of phosphate-solubilizing bacteria in the hyphosphere [J]. Journal of Experimental Botany, 2016, 67(6): 1689 − 1701. [118] JIANG Feiyan, ZHANG Lin, ZHOU Jiachao, et al. Arbuscular mycorrhizal fungi enhance mineralisation of organic phosphorus by carrying bacteria along their extraradical hyphae [J]. New Phytologist, 2021, 230(1): 304 − 315. [119] SINGH B K, NUNAN N, RIDGWAY K P, et al. Relationship between assemblages of mycorrhizal fungi and bacteria on grass roots [J]. Environmental Microbiology, 2008, 10(2): 534 − 541. [120] ZHANG Lin, PENG Yi, ZHOU Jiachao, et al. Addition of fructose to the maize hyphosphere increases phosphatase activity by changing bacterial community structure [J/OL]. Soil Biology and Biochemistry, 2020, 142: 107724[2022-11-15]. doi: 10.1016/j.soilbio.2020.107724. [121] ZHANG Lin, XU Minggang, LIU Yu, et al. Carbon and phosphorus exchange may enable cooperation between an arbuscular mycorrhizal fungus and a phosphate-solubilizing bacterium [J]. New Phytologist, 2016, 210(3): 1022 − 1032. [122] BEURA K, PRADHAN A K, GHOSH G K, et al. Root architecture, yield and phosphorus uptake by rice: response to rock phosphate enriched compost and microbial inoculants [J]. International Research Journal of Pure and Applied Chemistry, 2020, 21(19): 33 − 39. [123] LIU Junying, LIU Xuanshuai, ZHANG Qianbin, et al. Response of alfalfa growth to arbuscular mycorrhizal fungi and phosphate-solubilizing bacteria under different phosphorus application levels [J/OL]. AMB Express, 2020, 10(1): 200[2022-11-15]. doi: 10.1186/s13568-020-01137-w. [124] 付晓峰, 张桂萍, 张小伟, 等. 溶磷细菌和丛枝菌根真菌接种对南方红豆杉生长及根际微生物和土壤酶活性的影响[J]. 西北植物学报, 2016, 36(2): 353 − 360. FU Xiaofeng, ZHANG Guiping, ZHANG Xiaowei, et al. Effects of PSB and AMF on growth, microorganisms and soil enzyme activities in the rhizosphere of Taxus chinensis var. mairei seedlings [J]. Acta Botanica Boreali-Occidentalia Sinica, 2016, 36(2): 353 − 360. [125] BATTINI F, GRØNLUND M, AGNOLUCCI M, et al. Facilitation of phosphorus uptake in maize plants by mycorrhizosphere bacteria [J/OL]. Scientific Reports, 2017, 7(1): 4686[2022-11-15]. doi: 10.1038/s41598-017-04959-0. [126] 秦芳玲, 田中民. 同时接种解磷细菌与丛枝菌根真菌对低磷土壤红三叶草养分利用的影响[J]. 西北农林科技大学学报(自然科学版), 2009, 37(6): 151 − 157. QIN Fangling, TIAN Zhongmin. Effect of co-inoculation with arbuscular mycorrhizal fungi and four different phosphate-solubilizing bacteria on nutrients uptake of red clover in a low phosphorus soil [J]. Journal of Northwest A&F University (Nature Science Edition), 2009, 37(6): 151 − 157. [127] GARBAYE J. Helper bacteria-a new dimension to the mycorrhizal symbiosis [J]. New Phytologist, 1994, 128: 197 − 210. [128] AZCÓN-AGUILAR C, BAREA J M. Nutrient cycling in the mycorrhizosphere [J]. Journal of Soil Science and Plant Nutrition, 2015, 15(2): 372 − 396. [129] BURKE D J, KRETZER A M, RYGIEWICZ P T, et al. Soil bacterial diversity in a loblolly pine plantation: influence of ectomycorrhizas and fertilization [J]. FEMS Microbiology Ecology, 2006, 57: 409 − 419. [130] REDECKER D, SCHÜSSLER A, STOCKINGER H, et al. An evidence-based consensus for the classification of arbuscular mycorrhizal fungi (Glomeromycota) [J]. Mycorrhiza, 2013, 23(7): 515 − 531. [131] ETESAMI H, JEONG B R, GLICK B R. Contribution of arbuscular mycorrhizal fungi, phosphate-solubilizing bacteria, and silicon to P uptake by plant [J]. Frontiers in Plant Science, 2021, 12: 699618[2022-11-15]. doi: 10.3389/fpls.2021.699618. [132] WANG Yu, ZHANG Wenze, LIU Weikang, et al. Auxin is involved in arbuscular mycorrhizal fungi-promoted tomato growth and NADP-malic enzymes expression in continuous cropping substrates [J/OL]. BMC Plant Biology, 2021, 21(1): 48[2022-11-15]. doi: 10.1186/s12870-020-02817-2. [133] DELAUX P M, SCHORNACK S. Plant evolution driven by interactions with symbiotic and pathogenic microbes [J/OL]. Science, 2021, 371(6351): eaba6605[2022-11-15]. doi: 10.1126/science.aba6605. [134] DUAN Shilong, DECLERCK S, FENG Gu, et al. Hyphosphere interactions between Rhizophagus irregularis and Rahnella aquatilis promote carbon-phosphorus exchange at the peri-arbuscular space in Medicago truncatula [J]. Environmental Microbiology, 2023, 25(4): 867 − 879. [135] ZHANG Lin, FENG Gu, DECLERCK S. Signal beyond nutrient, fructose, exuded by an arbuscular mycorrhizal fungus triggers phytate mineralization by a phosphate solubilizing bacterium [J]. The ISME Journal, 2018, 12: 2339 − 2351. [136] WANG Letian, ZHANG Lin, GEORGE T S, et al. A core microbiome in the hyphosphere of arbuscular mycorrhizal fungi has functional significance in organic phosphorus mineralization [J]. New Phytologist, 2023, 238(2): 859 − 873. [137] 张林. AM真菌与解磷细菌相互作用提高有机磷利用效率的机理 [D]. 北京: 中国农业大学, 2016. ZHANG Lin. The Hyphosphere Interactions Between an Arbuscular Mycorrhiza Fungus and Phosphate Solubilizing Bacteria in Relation to Improving Organic Phosphate Utilization [D]. Beijing: China Agricultural University, 2016. [138] YAMAMOTO K, HIRAO K, OSHIMA T, et al. Functional characterization in vitro of all two-component signal transduction systems from Escherichia coli [J]. Journal of Biological Chemistry, 2005, 280(2): 1448 − 1456. [139] DUAN Shilong, DECLERCK S, ZHANG Lin, et al. Two-component system in Rahnella aquatilis is impacted by the hyphosphere of the arbuscular mycorrhizal fungus Rhizophagus irregularis [J]. Environmental Microbiology Reports, 2022, 14(1): 119 − 129. [140] ZHANG Lin, FAN Jiequn, FENG Gu, et al. The arbuscular mycorrhizal fungus Rhizophagus irregularis MUCL 43194 induces the gene expression of citrate synthase in the tricarboxylic acid cycle of the phosphate-solubilizing bacterium Rahnella aquatilis HX2 [J]. Mycorrhiza, 2019, 29(1): 69 − 75. [141] CHAUDHARY V B, HOLLAND E P, CHARMAN-ANDERSON S, et al. What are mycorrhizal traits? [J]. Trends in Ecology &Evolution, 2022, 37(7): 573 − 581. -
-
链接本文:
https://zlxb.zafu.edu.cn/article/doi/10.11833/j.issn.2095-0756.20220765
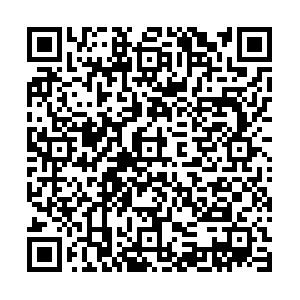
计量
- 文章访问数: 842
- HTML全文浏览量: 87
- PDF下载量: 246
- 被引次数: 0