-
碳、氮、磷是生命体构建的基础元素,通过微生物、植物、动物等的作用在土壤、大气和水等不同环境中相互转换、储存,形成了碳、氮、磷的生物地球化学循环[1−2]。在微生物驱动的生物地球化学循环过程中,几乎所有的生化反应都是酶促反应[3]。土壤胞外酶是指土壤介质中存在于细胞之外的各类酶的总称,通过微生物分泌或残体分解作用释放至胞外[4]。土壤胞外酶在土壤有机转化、氮磷元素循环等过程中发挥着至关重要的作用,是土壤关键元素循环的重要驱动力[5−6]。此外,土壤胞外酶分泌及活性受土壤养分有效性的调控,可作为不同生态系统养分循环与供应状况的重要指标[7−9]。
大气中二氧化碳(CO2)和其他温室气体的增加正导致全球变暖、降水模式改变以及氮沉降的增加,这些气候变化过程通过影响土壤性质对土壤的可持续性造成额外的威胁[10]。由于酶在自然环境中的活动受非生物因素(如温度、水分、pH等)和生物过程(如酶的合成和分泌等)控制,它们很可能对气候变化做出反映[11]。这些变化将对生态系统功能产生重要的影响,如有机质分解、养分循环和植物群落相互作用,最终将影响生产力和净碳平衡[12−13]。有关土壤胞外酶活性的研究集中于评估土壤碳储存、氮磷矿化转化等功能对土壤扰动、环境因素与管理变化的响应[14−17]。因此,在全球环境不断变化的背景下(气温升高、降水格局改变、氮沉降增加等),研究与碳、氮、磷循环相关的土壤胞外酶活性对气候变化的响应,对于深入了解全球变化背景下的土壤环境动态变化,明确全球变化对土壤碳、氮、磷循环的影响,以及评价各生态系统的可持续发展具有重要意义[18−21]。
近年来,通过土壤碳、氮、磷胞外酶活性计算得出的生态酶化学计量比已被广泛用于揭示不同生态系统的微生物资源限制。研究表明:在全球尺度上,碳、氮和磷获得酶的比值趋于1∶1∶1[22]。这表明土壤胞外酶活性分布具有一个共同的模式,但在各生态系统中土壤胞外酶化学计量比表现出差异[23]。如PENG等[24]利用生态酶化学计量理论揭示了草地土壤微生物的养分限制,表明草地微生物主要受氮的限制,其次受磷的限制。XU等[25]利用生态酶化学计量比揭示了热带森林土壤微生物主要受磷的限制。揭示生态环境中的微生物资源限制,对于提高生态系统生产力、养分循环等方面具有重要意义[26]。但是,在当今气候变化逐渐加剧的背景下,仍缺乏气候变化对生态酶化学计量比影响规律的总结。
本研究基于碳、氮、磷生物地球化学循环过程,总结相关土壤胞外酶的来源、类型及其在不同生态系统中对气候变化的响应,着重分析生态酶化学计量学的研究进展,并在此基础上明确了气候变化背景下土壤胞外酶的关键研究点。
-
土壤胞外酶由土壤微生物、植物及无脊椎动物产生。其中,细菌和真菌是土壤胞外酶的主要来源,土壤胞外酶的主要作用是催化降解复杂有机质为有机单体或矿物质营养元素,以供微生物生长繁殖[27]。在碳循环酶中,真菌中的白腐菌和褐腐菌是木质素分解酶系的主要生产者,其次植物根系也会产生漆酶降解木质素[28];纤维素分解酶系主要由真菌(木霉属Trichoderma、腐霉属Pythium)与细菌(链霉菌Streptomyces、芽孢杆菌属Bacillus)产生[29]。同时,微生物也是氮循环酶的主要来源。如链霉菌可以产生N-乙酰氨基葡萄糖苷酶和壳多糖酶;芽孢杆菌是许多高效碱性蛋白酶的主要来源[30]。对于与磷循环相关的酶而言,酸性磷酸单酯酶与磷酸酶是由包括细菌、原生动物、菌根真菌和腐生真菌在内的各种生物在植物根际土壤中产生的,另外植物根系分泌物也是其重要来源[31]。
近几十年来,基因组学(宏基因组)的发展为探明基因表达和酶活性之间的相关性成为可能,这为寻找土壤胞外酶的特定来源提供了路径[32]。如KELLNER等[33]在森林土壤中获得了产生木质素酶的真菌和细菌基因,并强调了它们在土壤碳循环中的关键作用。但由于土壤的复杂性和酶编码基因的PCR引物设计困难,只有少数研究探明了功能基因丰度和土壤酶活性之间的关系[34]。其次,与土壤酶活性相关的土壤转录组学和蛋白质组学仍处于起步阶段,有关分子技术在土壤酶的研究中应用较少[35]。随着分子生物学技术和同位素示踪等方法的改进,有关土壤酶来源和作用路径的研究必将取得新的突破。
-
国际上最常用的土壤酶分类体系是按照酶功能将土壤酶划分为氧化还原酶、水解酶、转移酶、裂合酶、连接酶和异构酶。参与土壤碳、氮、磷循环的土壤胞外酶主要归属于水解酶与氧化还原酶[36]。
土壤水解酶是参与催化土壤大分子物质进行水解反应的一类酶,其活性直接影响纤维素、半纤维素等易于分解的有机物降解[37]。如纤维素酶催化水解纤维素为低聚糖,而后β-葡萄糖苷酶催化低聚糖水解并释放葡萄糖[38]。氧化还原酶则是指催化土壤中的各种底物发生氧化还原反应酶类的统称,氧化还原酶与木质素等不易分解的有机物降解以及溶解性有机碳生成密切相关[39]。如多酚氧化酶参与木质素以及腐殖质在内的多种酚类化合物的转化,催化多环芳烃降解为醌类代谢物,为微生物提供碳和能量[40]。此外,根据土壤胞外酶参与的养分循环将其土壤酶划分为土壤碳循环酶、氮循环酶及磷循环酶[41]。土壤碳、氮、磷循环酶分别参与土壤碳、氮、磷循环的周转,为微生物与植物释放可吸收碳源、氮源及磷源的胞外酶。常见与土壤碳循环过程相关的土壤胞外酶包括纤维素分解酶、淀粉酶、蔗糖酶、木质素分解酶、多糖降解酶等;氮循环过程相关的酶有硝化酶、氨同化酶、生物固氮酶以及脲酶、蛋白酶等氮同化酶;磷循环过程相关的酶有磷酸酶、磷酸二酯酶等[42]。
-
微生物通过调控胞外酶的产生以适应土壤养分资源的限制,因此参与养分循环的土壤胞外酶活性常用于指示微生物的养分需求[43]。1994年,SZINSABAUGH[44]基于养分获取策略首次提出生态酶化学计量理论。该理论利用从土壤环境中释放可同化养分(碳、氮和磷)的几个关键胞外酶活性比值,预测碳、氮、磷的相对有效性,揭示土壤微生物的资源限制[45−46]。常用的酶包括碳酶葡萄糖苷酶(BG)、氮酶乙酰氨基葡糖苷酶(NAG)、亮氨酸氨基肽酶(LAP)、磷酶磷酸酶(AP)[47]。BG、(NAG+LAP)和AP分别表示土壤碳、氮和磷的有效性[48]。BG/(NAG+LAP)、BG/AP和(NAG+LAP)/AP是指微生物C/N、C/P和N/P利用。当BG/(NAG+LAP)和BG/AP<1.0时,意味着相对于微生物碳的氮或磷限制;当(NAG+LAP)/AP<1.0时,意味着微生物磷相对于氮限制,反之亦然[49−50]。该理论被应用于林地、草地、亚热带湿地等不同生态系统[51−53],对进一步理解碳、氮、磷生物地球化学化学过程相关的土壤胞外酶的产生和微生物养分获取提供了理论支持。
为识别碳、氮、磷酶同时存在时微生物的相对资源需求,MOORHEAD等[54]于2016年提出微生物养分限制的向量分析方法(长度和角度),将碳、氮、磷酶比值转换为向量长度和角度,提供了相对碳限制(向量长度)和相对氮、磷限制(向量角度)的明确度量,以量化微生物对碳的获取以及氮、磷获取的相对投入,表征微生物的代谢特征。
但在气候变化情景下,微生物所需的土壤碳、氮、磷源特征将改变,为了更好地探讨气候变化背景下土壤碳、氮、磷相关的生物地球化学循环,需进一步改进生态酶化学计量比的计算方法和理论。微生物通过多种酶作用于不同底物(如纤维素、木质素、蛋白质、甲壳素、氨基酸等)来获取养分,分解各种酶参与碳、氮、磷的生物地球化学过程[12]。而生态酶化学计量理论涉及到的土壤胞外酶是以分解木质纤维素为主,这些水解酶可能不足以准确指示微生物多渠道的养分获取策略[38]。为此,结合MORI[55]、CUI等[56]对生态酶化学计量理论及模型的改进,未来需根据不同养分来源(纤维素、木质素、蛋白质、甲壳素、氨基酸等)为微生物供给养分的比例,给催化不同底物的酶赋予权重,整合多种酶进行计算,进一步揭示气候变化背景下,土壤酶对碳、氮、磷循环过程的贡献。
-
近年来,大气氮沉降以不同形态输入土壤(硝态氮、铵态氮、有机氮),因此需要归纳无机氮、有机氮对不同生态系统土壤胞外酶活性的影响[57]。总的来说,草地土壤胞外酶活性对不同氮的输入类型(无机氮、有机氮)响应一致,即氮的输入抑制了氮酶活性,刺激了碳酶、磷酶活性[58−60]。氮肥输入增加了土壤氮的有效性,在土壤环境氮素富集增加的同时,转变为碳和磷受限的情况。根据“资源配置理论”,微生物更倾向于吸收利用容易获得的氮元素,将更多的能量分配于产生其他类型的胞外酶获得其他营养元素(碳和磷),达到能量分配的最优化[61]。因此,施氮引起的土壤养分化学计量比变化与氮有效性的增加是改变土壤胞外酶活性的主要原因。而且氮的添加使土壤pH降低,影响土壤微生物组成与分布,从而间接影响了土壤胞外酶活性[62]。 然而,在物质循环周转较快的热带森林,氮肥的添加对土壤碳、氮循环酶影响并不显著[63−65]。
导致森林土壤胞外酶活性对氮输入响应与其他生态系统不一致的原因可能是养分限制的差异。不同生态系统,如热带森林与温度草地的养分限制是由气候差异以及自身养分循环特点所决定[66]。在高度风化的热带土壤中,磷随矿物风化和土壤发育逐渐减少,而氮可由生物固氮和氮沉积随着时间推移而积累[67]。并且在潮湿的热带森林中,充足的降水可能增加了淋溶而进一步降低磷的有效性[68]。相比之下,大部分草原寒冷和干燥的气候减缓了生物固氮和矿化速率[69]。这导致了热带森林的磷限制强于草地,氮限制则相反。因此在磷限制的森林土壤中加氮可能是多余的,而且森林土壤理化性质及微生物群落结构对氮输入的响应均不显著,这表明了森林对氮输入具有一定抗性;另一方面,氮的添加可能会被树木快速吸收,由此导致酶活性并无改变[70]。因此,氮沉降预计将对氮限制的草地生态系统产生更大的影响。
氮的输入改变了土壤胞外酶化学计量比及矢量特征,表明氮输入影响了微生物的养分限制。通过生态酶化学计量比及矢量分析、相关田间试验及Meta分析,发现氮输入加重了微生物碳、磷限制[71−73]。氮的添加会增加凋落物氮、磷含量,以及导致土壤酸化降低根系分泌物,从而进一步降低土壤有效碳含量[74]。另一方面,氮的添加延缓了有机质的矿化,导致微生物对碳需求的增加[75]。微生物磷限制的加剧归因于施氮促进了植物生长,进而增加了植物对磷的需求和吸收,加剧了植物与微生物的养分竞争[76]。但在一些研究中,施氮并没有影响土壤胞外酶化学计量比及矢量分析,表明氮的添加并没有加剧磷的限制[77]。因此,氮输入对微生物磷限制的影响机制仍需探讨,还需明确氮添加导致的调节氮、磷有效性相关的微生物及其资源之间化学计量关系,进而获得氮添加对不同生态系统土壤胞外酶定量化影响的调控机制。
-
温度和水分是影响土壤酶活性的2个关键非生物因子,全球变暖和全球降水格局的改变都会对土壤酶活性产生复杂的作用[78]。不同生态系统中的土壤胞外酶活性对土壤温度增加的响应与适应特征差异显著[79−80]。从时间尺度上来说,大多数生态环境中(森林、草地、湿地等)短期内的土壤温度增加会导致微生物活性与微生物量的增加,进而增加土壤胞外酶活性[81−82]。但随着时间的推移,土壤温度增加可能导致土壤胞外酶的生产和分泌减少以及降低底物与酶的亲和力以此抵消酶活性的升高[83]。因此,土壤胞外酶活性在不同生态系统对增温时间长短有共同的响应与适应动态。其次土壤胞外酶活性对于土壤温度增加的响应也取决于酶的种类与增温幅度,不同土壤胞外酶活性对增温响应存在差异,这可能是不同土壤胞外酶对温度敏感性不同所致[84]。即使处于高温条件,胞外酶也可以在土壤环境中积累,因此土壤环境中可能存在一个酶库,使土壤微生物适应温度变化,土壤胞外酶活性对季节性的温度变化响应显著也证明了这一点[85]。相关研究表明:增温所导致的土壤碳储量减少与木质素酶活性/纤维素酶活性的增加显著相关,也就是说长期的气候变暖刺激了微生物对土壤碳库的利用[86]。可见,未来更需关注气候变暖如何通过影响土壤胞外酶活性改变生态系统功能的机制。
土壤温度升高引起土壤养分周转、微生物活性改变,从而影响土壤胞外酶化学计量比。ZHENG等[87]研究发现:土壤短期增温降低了热带森林土壤微生物的碳限制,增加了微生物的磷限制,这与GUAN等[88]的研究一致。碳限制的缓解可能与土壤可利用碳的积累随着温度升高而增加有关,从而减少了微生物对碳的需求[89]。另外一些研究表明:温度升高可加速细胞膜电荷维持与脂质周转,增加了微生物对氮、磷的需求[90]。然而,相关研究表明:土壤长期增温对土壤胞外酶化学计量比的影响并不显著,也就是说长期增温并不影响土壤碳、氮相关微生物代谢[91]。其原因可能是长期增温后土壤微生物已经适应了环境的变化,改变了自身组成与功能,因而降低了胞外酶对温度的敏感性[92]。
-
全球降水格局的改变影响着土壤水分的有效性,间接影响土壤性质与结构(团聚体、pH),进而影响土壤养分有效性[93]。这些改变可能进一步刺激微生物生长和生理活性,影响土壤胞外酶的合成以及改变微生物的资源限制[94]。土壤含水率与质地决定了根系分泌物的释放以及胞外酶在基质与反应产物之间的扩散速率及亲和力[95]。适当增加土壤水分,土壤胞外酶活性会相应增加[96−97]。而土壤水分过低或过高都会降低胞外酶活性[98]。土壤微生物对酶的分配具有一定的气候调节作用,总的来说土壤胞外酶活性对于降水改变的响应程度强于温度[99]。这是因为微生物组成受土壤含水率的影响,但是定量化胞外酶对降水的响应机制需结合宏基因组与定量PCR进行探讨。
降水增加对微生物碳限制与氮、磷限制的影响不同。CUI等[100]、LI等[101]利用土壤胞外酶化学计量比及矢量特征,揭示了干旱地区增加降水减弱了土壤微生物的碳限制。植物受到土壤湿度增加的刺激而生长,通过增加植物残基向微生物提供足够的碳输入,减少了碳获取酶的分泌[102]。而降水对土壤微生物氮、磷的限制在干旱、潮湿地区表现出不同。LI等[103]用土壤胞外酶化学计量比及矢量特征揭示不同降水梯度对微生物养分限制的影响,表明在干旱地区,降水增加驱使土壤微生物从磷限制向氮限制转变,在潮湿地区反而加重了微生物的磷限制。
-
CO2浓度增加刺激了碳、氮酶活性,对磷酶活性影响不显著。田间试验表明:CO2浓度升高刺激了氮酶(β-乙酰氨基葡萄糖苷酶)活性,特别是在氮限制的温带森林和草地土壤中[104]。CO2浓度升高增加了根系生物量以及根系分泌物的输入,根系的养分周转速率加快,提高了氮酶活性和氮周转速率,以便满足植物及微生物对氮的需求[105]。对于碳酶而言,其活性对CO2浓度升高的响应与持续时间相关。FINZI等[106]研究发现:β-葡萄糖苷酶、纤维素酶活性在CO2浓度升高的刺激下增加,这可以归因于根源有机质输入的增加,但在CO2浓度升高5 a后酚氧化酶活性才显著增加,FANG等[107]研究同样表明:CO2浓度升高刺激酚氧化酶活性。这表明随着时间的推移,微生物群落对碳的需求增加,这可能是激发效应的结果,木质素酶活性的增加促进了难降解碳的分解,增加了碳的周转和损失,这可能导致土壤碳库进一步降低[108−109]。磷酸酶活性对CO2浓度升高并不敏感,OCHOA-HUESO等[110]研究表明:在磷限制的森林中,CO2浓度的升高并没有改变磷酸酶活性。磷酸酶活性的不敏感可能是由于CO2浓度升高刺激了碳、氮酶活性增加进而促进了碳、氮循环的周转,这会促进磷的活化程度以及提高土壤磷的有效性[111]。并且CO2浓度升高导致的根系碳渗出物(螯合剂)可能对矿物磷的溶解具有重要作用[112]。然而,随着植物和微生物生长发育,可能导致土壤有效磷含量降低,土壤磷酸酶活性是否发生变化仍不确定。而且,采用土壤胞外酶化学计量比对CO2富集响应的相关研究仍然较少,尚不清楚CO2浓度升高会不会影响微生物养分限制模式。为充分了解生态系统对CO2浓度上升的响应,以整合到生物地球化学模型中,仍需增加田间试验时长,开展长期模拟CO2浓度升高(FACE试验)的研究。
-
气候变化因子(包括增温、降水改变、氮沉降、CO2浓度升高等)是影响土壤碳储存等生态功能的重要环境扰动因素,土壤碳、氮、磷酶活性对气候变化的响应既具有生态系统的共性,也具有特异性。各生态系统的养分限制是导致土壤胞外酶活性对养分输入产生生态特异性的主要因素。为此,在今后的研究中应更加关注养分输入所引起的土壤生态功能的变化与土壤胞外酶活性变化之间的作用机制,以期在气候变化背景下,预测各因子对生态环境功能的影响。在气候变化的背景下,土壤胞外酶活性在土壤中似乎受到更加复杂因素的调控,土壤胞外酶活性对于气候变化的响应机制尚不清楚,表现在没有统一的量度衡量气温升高与降水改变对土壤胞外酶活性的影响。未来应注重研究气温、降水与其他因素的交互作用对土壤胞外酶活性的影响,整合多种土壤胞外酶活性的化学计量指标探讨碳氮、磷等元素的生物地球化学循环过程。
土壤是一个复杂的生态系统,处在动态平衡当中,将特定的土壤微生物功能类群与碳、氮、磷代谢过程相关的土壤胞外酶定量联系起来是目前土壤酶学研究的难点与重点。土壤胞外酶与土壤微生物活性、微生物量与微生物功能基因丰度等密切相关,理解微生物如何调控土壤胞外酶合成、分泌以及存活的机制是预测全球变化背景下土壤碳、氮、磷生物地球化学过程的关键。为此,需基于宏基因组、转录组及代谢组的技术了解微生物群落结构与功能之间的联系,结合稳定性同位素探针技术(stable isotope probing,SIP技术)在DNA水平(DNA-SIP技术)与RNA水平(RNA-SIP技术)量化不同微生物功能类群与特定土壤胞外酶的定量化关系,明确气候变化背景下,土壤胞外酶对土壤碳、氮、磷生物地球化学过程的调控机制,为进一步完善碳、氮、磷生物地球化学模型提供依据。
Research progress in the response of soil extracellular enzymes activity to climate changes
-
摘要: 土壤胞外酶在驱动生态系统碳、氮、磷等元素的生物地球化学循环中发挥着不可或缺的作用。在全球变化背景下,揭示土壤胞外酶活性对气候变化的响应可促进有关碳、氮、磷生态系统关键过程对气候变化响应的认识,但目前不同生态系统碳、氮、磷相关的土壤胞外酶活性对气候变化响应机制的认识程度仍需加强。本研究综述了参与碳、氮、磷生物地球化学过程的土壤胞外酶的来源、分类及调控因子,并且对土壤胞外酶参与的生物地球化学过程进行了综述。在此基础上,阐述了土壤胞外酶活性对氮沉降、增温、降水及二氧化碳(CO2)变化的响应规律及机制。总结了生态酶化学计量对常见全球变化因子的响应机制,基于近年来对完善生态酶化学计量理论的探讨,提出了此理论发展的新角度。为此,在全球变化背景下需结合代谢组与分子生物学加强土壤胞外酶对气候变化响应机制的研究。本研究可为进一步理解气候变化情景下,土壤胞外酶在生物地球化学循环中的作用提供了新视角。参112Abstract: Soil extracellular enzymes play indispensable role in the driving of biogeochemical cycle of carbon, nitrogen, phosphorus and other elements in soil ecosystem. Therefore, under the background of global changes, disclosing the response of soil extracellular enzyme activity to variation of climate can improve the understanding of the mechanisms of soil carbon, nitrogen and phosphorus key biogeochemical processes under climate change. However, the current understanding of the response mechanism of soil extracellular enzyme activities related to carbon, nitrogen and phosphorus in different ecosystems to climate change still needs to strengthen. Thus, the sources, classification and regulatory factors of soil extracellular enzymes in the biogeochemical cycle of carbon, nitrogen and phosphorus, and the biogeochemical processes of soil extracellular enzymes were summarized. On this basis, the response rules and mechanisms of soil extracellular enzyme activity to nitrogen deposition, warming, precipitation and CO2 changes were discussed. The response mechanism of soil extracellular enzyme stoichiometry to common global change factors was summarized. Therefore, in the context of global change, it is necessary to combine metabolome and molecular biology to strengthen the response mechanism of soil extracellular enzymes to climate change. This review provides a new perspective for further understanding the role of soil extracellular enzymes in biogeochemical cycles under climate change scenarios. [Ch, 112 ref.]
-
[1] DING Wenli, CONG Wenfeng, LAMBERS H. Plant phosphorus-acquisition and -use strategies affect soil carbon cycling [J]. Trends in Ecology &Evolution, 2021, 36(10): 899 − 906. [2] ACHAT D L, AUGUSTO L, GALLET-BUDYNEK A, et al. Future challenges in coupled C-N-P cycle models for terrestrial ecosystems under global change: a review [J]. Biogeochemistry, 2016, 131(1/2): 173 − 202. [3] ACOSTA-MARTINEZ V, CANO A, JOHNSON J. Simultaneous determination of multiple soil enzyme activities for soil health-biogeochemical indices [J]. Applied Soil Ecology, 2018, 126: 121 − 128. [4] LUO Ling, MENG Han, GU Jidong. Microbial extracellular enzymes in biogeochemical cycling of ecosystems [J]. Journal of Environmental Management, 2017, 197: 539 − 549. [5] AMEUR D, ZEHETNER F, JOHNEN S, et al. Activated biochar alters activities of carbon and nitrogen acquiring soil enzymes [J]. Pedobiologia, 2018, 69: 1 − 10. [6] ATTADEMO A M, SANCHEZ-HERNANDEZ J C, LAJMANOVICH R C, et al. Enzyme activities as indicators of soil quality: response to intensive soybean and rice crops [J/OL]. Water, Air, & Soil Pollution, 2021, 232: 295[2022-09-20]. doi:10.1007/s11270-021-05211-2. [7] CHEN Xin, LUO Min, LIU Yuxiu, et al. Linking carbon-degrading enzyme activity to microbial carbon-use trophic strategy under salinization in a subtropical tidal wetland [J/OL]. Applied Soil Ecology, 2022, 174: 11[2022-09-20]. doi:10.1016/J.APSOIL.2022.104421. [8] CENINI V L, FORNARA D A, MCMULLAN G, et al. Linkages between extracellular enzyme activities and the carbon and nitrogen content of grassland soils [J]. Soil Biology and Biochemistry, 2016, 96: 198 − 206. [9] AKHTAR K, WANG Weiya, KHAN A, et al. Straw mulching with fertilizer nitrogen: an approach for improving crop yield, soil nutrients and enzyme activities [J]. Soil Use and Management, 2019, 35(3): 526 − 535. [10] ZHANG Pingjiu, LI Lianqing, PAN Genxing, et al. Soil quality changes in land degradation as indicated by soil chemical, biochemical and microbiological properties in a karst area of southwest Guizhou, China [J]. Environmental Geology, 2006, 51(4): 609 − 619. [11] ALLISON S D. Cheaters, diffusion and nutrients constrain decomposition by microbial enzymes in spatially structured environments [J]. Ecology Letter, 2005, 8(6): 626 − 635. [12] CUI Yongxing, WANG Xia, WANG Xiangxiang, et al. Evaluation methods of heavy metal pollution in soils based on enzyme activities: a review [J]. Soil Ecology Letters, 2021, 3(3): 169 − 177. [13] de BARROS J A, de MEDEIROS E V, da COSTA D P, et al. Human disturbance affects enzyme activity, microbial biomass and organic carbon in tropical dry sub-humid pasture and forest soils [J/OL]. Archives of Agronomy and Soil Science, 2019[2022-09-20]. doi: 10.1080/03650340.2019.1622095. [14] BASTIDA F, SELEVSEK N, TORRES I F, et al. Soil restoration with organic amendments: linking cellular functionality and ecosystem processes [J/OL]. Scientific Reports, 2015, 5[2022-09-20]. doi: 10.1038/srep15550. [15] GRANDY A S, STRICKLAND M S, LAUBER C L, et al. The influence of microbial communities, management, and soil texture on soil organic matter chemistry [J]. Geoderma, 2009, 150(3/4): 278-286. [16] HENDRIKSEN N B, CREAMER R E, STONE D, et al. Soil exo-enzyme activities across Europe-the influence of climate, land-use and soil properties [J]. Applied Soil Ecology, 2016, 97: 44 − 48. [17] ANANBEH H, STOJANOVIC M, POMPEIANO A, et al. Use of soil enzyme activities to assess the recovery of soil functions in abandoned coppice forest systems [J/OL]. Science of the Total Environment, 2019, 694: 133692[2022-09-20]. doi. org/10.1016/j. scitotenv. 2019.133692. [18] FINZI A C, AUSTIN A T, CLELAND E E, et al. Responses and feedbacks of coupled biogeochemical cycles to climate change: examples from terrestrial ecosystems [J]. Frontiers in Ecology and the Environment, 2011, 9(1): 61 − 67. [19] XIAO Wen, CHEN Xiao, JING Xin, et al. A meta-analysis of soil extracellular enzyme activities in response to global change [J]. Soil Biol Biochem, 2018, 123: 21 − 32. [20] CHEN Ji, LUO Yiqi, VAN GROENIGEN K J, et al. A keystone microbial enzyme for nitrogen control of soil carbon storage [J/OL]. Science Advances, 2018, 4(8): 1689[2022-09-20]. doi: 10.1126/sciadv.aaq1689. [21] LEE S H, KIM M S, KIM J G, et al. Use of soil enzymes as indicators for contaminated soil monitoring and sustainable management [J/OL]. Sustainability, 2020, 12(19): 8209[2022-09-20]. doi: 10.3390/su12198209. [22] SINSABAUGH R L, LAUBER C L, WEINTRAUB M N, et al. Stoichiometry of soil enzyme activity at global scale [J]. Ecology Letters, 2008, 11: 1252 − 1264. [23] ZHOU Luhong, LIU Shangshi, SHEN Haihua, et al. Soil extracellular enzyme activity and stoichiometry in China’s forests [J]. Functional Ecology, 2020, 34(7): 1461 − 1471. [24] PENG Xiaoqian, WANG Wei. Stoichiometry of soil extracellular enzyme activity along a climatic transect in temperate grasslands of northern China [J]. Soil Biology and Biochemistry, 2016, 98: 74 − 84. [25] XU Zhiwei, YU Guirui, ZHANG Xinyu, et al. Soil enzyme activity and stoichiometry in forest ecosystems along the North-South Transect in eastern China (NSTEC) [J]. Soil Biology and Biochemistry, 2017, 104: 152 − 163. [26] ZHANG Qian, FENG Jiao, WU Junjun, et al. Variations in carbon-decomposition enzyme activities respond differently to land use change in central China [J]. Land Degradation Development, 2019, 30(4): 459 − 469. [27] FUHRMAN J A. Microbial community structure and its functional implications [J]. Nature, 2009, 459: 193 − 199. [28] BURKE R M, CAIRNEY J W G. Laccases and other polyphenol oxidases in ecto- and ericoid mycorrhizal fungi [J]. Mycorrhiza, 2002, 12: 105 − 116. [29] ASGHAR W, KATAOKA R. Effect of co-application of Trichoderma spp. with organic composts on plant growth enhancement, soil enzymes and fungal community in soil [J]. Archives of Microbiology, 2021, 203: 4281 − 4291. [30] BALUME I, AGUMAS B, MUSYOKI M, et al. Potential proteolytic enzyme activities modulate archaeal and bacterial nitrifier abundance in soils differing in acidity and organic residue treatment [J/OL]. Applied Soil Ecology, 2022, 169: 104188[2022-09-20]. doi: 10.1016/j.apsoil.2021.104188. [31] GHORBANI-NASRABADI R, GREINER R, ALIKHANI H A, et al. Distribution of actinomycetes in different soil ecosystems and effect of media composition on extracellular phosphatase activity [J]. Journal Soil Science and Plant Nutrition, 2013, 13(1): 223 − 236. [32] CALDWELL B A. Enzyme activities as a component of soil biodiversity: a review [J]. Pedobiologia, 2005, 49(6): 637 − 644. [33] KELLNER H, LUIS P, SCHLITT B, et al. Temporal changes in diversity and expression patterns of fungal laccase genes within the organic horizon of a brown forest soil [J]. Soil Bioogyl Biochemistry, 2009, 41(7): 1380 − 1389. [34] LUIS P, WALTHER G, KELLNER H, et al. Diversity of laccase genes from basidiomycetes in a forest soil [J]. Soil Biology and Biochemistry, 2004, 36(7): 1025 − 1036. [35] LI Minghao, HE Wei, GU Jidong. Enhanced plant-microbe remediation of PCBs in soil using enzyme modification technique combined with molecular docking and molecular dynamics [J]. Biochemical Journal, 2021, 478(10): 1921 − 1941. [36] NANNIPIERI P, GIAGNONI L, RENELLA G, et al. Soil enzymology: classical and molecular approaches [J]. Biology and Fertility of Soils, 2012, 48: 743 − 762. [37] BRACKIN R, ROBINSON N, LAKSHMANAN P, et al. Microbial function in adjacent subtropical forest and agricultural soil [J]. Soil Biology and Biochemistry, 2013, 57: 68 − 77. [38] 王理德, 王方琳, 郭春秀, 等. 土壤酶学硏究进展[J]. 土壤, 2016, 48(1): 12 − 21. WANG Lide, WANG Fanglin, GUO Chunxiu, et al. Review: progress of soil enzymology [J]. Soils, 2016, 48(1): 12 − 21. [39] RYE C S, WITHERS S G. Glycosidase mechanisms [J]. Current Opinion in Chemical Biology, 2000, 4(5): 573 − 580. [40] SINSABAUGH R L. Phenoloxidase, peroxidase and organic matter dynamics of soil [J]. Soil Biology and Biochemistry, 2010, 42(3): 391 − 404. [41] BAI Xuejuan, DIPPOLD M A, AN Shaoshan, et al. Extracellular enzyme activity and stoichiometry: the effect of soil microbial element limitation during leaf litter decomposition [J/OL]. Ecological Indicators, 2021, 121: 107200[2022-09-20]. doi: 10.1016/j.ecolind.2020.107200. [42] ADETUNJI A T, LEWU F B, MULIDZI R, et al. The biological activities of beta-glucosidase, phosphatase and urease as soil quality indicators: a review [J]. Journal Soil Science Plant Nutrition, 2017, 17(3): 794 − 807. [43] SCHIMEL J, BECERRA C A, BLANKINSHIP J. Estimating decay dynamics for enzyme activities in soils from different ecosystems [J]. Soil Biology and Biochemistry, 2017, 114: 5 − 11. [44] SZINSABAUGH R S. Enzymic analysis of microbial pattern and process [J]. Biology and Fertility of Soils, 1994, 17: 69 − 74. [45] EKENLER M, TABATABAI M A. β-glucosaminidase activity as an index of nitrogen mineralization in soils [J]. Communications in Soil Science and Plant Analysis, 2004, 35(7/8): 1081 − 1094. [46] FUJITA K, MIYABARA Y, KUNITO T. Microbial biomass and ecoenzymatic stoichiometries vary in response to nutrient availability in an arable soil [J]. European Journal of Soil Biology, 2019, 91: 1 − 8. [47] PENTON C R, NEWMAN S. Enzyme-based resource allocated decomposition and landscape heterogeneity in the Florida Everglades [J]. Journal of Environment Quality, 2008, 37(3): 972 − 976. [48] SINSABAUGH R L, FOLLSTAD SHAH J J. Ecoenzymatic stoichiometry of recalcitrant organic matter decomposition: the growth rate hypothesis in reverse [J]. Biogeochemistry, 2011, 102: 31 − 43. [49] KANTE M, RIAH W, CLIQUET J B, et al. Soil enzyme activity and stoichiometry: linking soil microorganism resource requirement and legume carbon rhizodeposition [J/OL]. Agronomyl, 2021, 11(11): 2131[2022-09-20]. doi: 10.3390/agronomy11112131. [50] SINSABAUGH R L, FOLLSTAD SHAH J J, HILL B H, et al. Ecoenzymatic stoichiometry of stream sediments with comparison to terrestrial soils [J]. Biogeochemistry, 2012, 111(1/3): 455 − 467. [51] CHEN Ruirui, SENBAYRAM M, BLAGODATSKY S, et al. Soil C and N availability determine the priming effect: microbial N mining and stoichiometric decomposition theories [J/OL]. Global Change Biology, 2014, 20[2022-09-20]. doi: 10.1111/gcb.12475. [52] WANG Jipeng, WU Yanhong, LI Jingji, et al. Soil enzyme stoichiometry is tightly linked to microbial community composition in successional ecosystems after glacier retreat [J/OL]. Soil Biology Biochemistry, 2021, 162: 108429[2022-09-20]. doi: 10.1016/j.soilbio.2021.108429. [53] WARING B G, WEINTRAUB S R, SINSABAUGH R L. Ecoenzymatic stoichiometry of microbial nutrient acquisition in tropical soils [J]. Biogeochemistry, 2014, 117(1): 101 − 113. [54] MOORHEAD D L, SINSABAUGH R L, HILL B H, et al. Vector analysis of ecoenzyme activities reveal constraints on coupled C, N and P dynamics [J]. Soil Biology and Biochemistry, 2016, 93: 1 − 7. [55] MORI T. Does ecoenzymatic stoichiometry really determine microbial nutrient limitations? [J/OL]. Soil Biology and Biochemistry, 2020, 146: 107816[2022-09-20]. doi: 10.1016/j.soilbio.2020.107816. [56] CUI Yongxing, MOORHEAD D L, GUO Xiaobin, et al. Stoichiometric models of microbial metabolic limitation in soil systems [J]. Global Ecology and Biogeography, 2021, 30(11): 2297 − 2311. [57] WANG Congyan, LÜ Yanna, LIU Xueyan, et al. Ecological effects of atmospheric nitrogen deposition on soil enzyme activity [J]. Journal of Forestry Research, 2013, 24(1): 109 − 114. [58] DONG Chengcheng, WANG Wei, LIU Hongyan, et al. Comparison of soil microbial responses to nitrogen addition between ex-arable grassland and natural grassland [J]. Journal of Soils and Sediments, 2021, 21(3): 1371 − 1384. [59] CHEN Ji, VAN GROENIGEN K J, HUNGATE B A, et al. Long-term nitrogen loading alleviates phosphorus limitation in terrestrial ecosystems [J]. Global Change Biology, 2020, 26(9): 5077 − 5086. [60] 勒佳佳, 苏原, 彭庆文, 等. 氮添加对天山高寒草原土壤酶活性和酶化学计量特征的影响[J]. 干旱区研究, 2020, 37(2): 382 − 389. LE Jiajia, SU Yuan, PENG Qingwen, et al. Effects of nitrogen addition on soil enzyme activities and ecoenzymatic stoichiometry in alpine grassland of the Tianshan Mountains [J]. Arid Zone Research, 2020, 37(2): 382 − 389. [61] VOURLITIS G L, KIRBY K, VALLEJO I, et al. Potential soil extracellular enzyme activity is altered by long-term experimental nitrogen deposition in semiarid shrublands [J/OL]. Applied Soil Ecology, 2021, 158: 103779[2022-09-20]. doi: 10.1016/j.apsoil.2020.103779. [62] YAN Bangguo, SUN Yi, HE Guangxiong, et al. Nitrogen enrichment affects soil enzymatic stoichiometry via soil acidification in arid and hot land [J/OL]. Pedobiologia, 2020, 81/82: 150663[2022-09-20]. doi: 10.1016/j.pedobi.2020.150663. [63] JING Xin, CHEN Xiao, TANG Mao, et al. Nitrogen deposition has minor effect on soil extracellular enzyme activities in six Chinese forests [J]. Science of the Total Environment, 2017, 607: 806 − 815. [64] MA Suhui, CHEN Guoping, TANG Wenguang, et al. Inconsistent responses of soil microbial community structure and enzyme activity to nitrogen and phosphorus additions in two tropical forests [J]. Plant and Soil, 2021, 460(1): 453 − 468. [65] TURNER B L, WRIGHT S J. The response of microbial biomass and hydrolytic enzymes to a decade of nitrogen, phosphorus, and potassium addition in a lowland tropical rain forest [J]. Biogeochemistry, 2014, 117(1): 115 − 130. [66] JING Xin, CHEN Xiao, FANG Jingyun, et al. Soil microbial carbon and nutrient constraints are driven more by climate and soil physicochemical properties than by nutrient addition in forest ecosystems [J/OL]. Soil Biology and Biochemistry, 2020, 141: 107657[2022-09-20]. doi:10.1016/j.soilbio.2019.107657. [67] MENGE D N L, PACALA S W, HEDIN L O. Emergence and maintenance of nutrient limitation over multiple timescales in terrestrial ecosystems [J]. American Naturalist, 2009, 173(2): 164 − 175. [68] MORI T, LU Xiankai, AOYAGI R, et al. Reconsidering the phosphorus limitation of soil microbial activity in tropical forests [J]. Functional Ecology, 2018, 32(5): 1145 − 1154. [69] CAMENZIND T, HATTENSCHWILER S, TRESEDER K K, et al. Nutrient limitation of soil microbial processes in tropical forests [J]. Ecological Monographs, 2018, 88(1): 4 − 21. [70] ULLAH S, AI Chao, HUANG Shaohui, et al. The responses of extracellular enzyme activities and microbial community composition under nitrogen addition in an upland soil [J/OL]. PLoS One, 2019, 14(9): e0223026[2022-09-20]. doi: 10.1371/journal.pone.0223026. [71] MA Wenjun, LI Jian, GAO Ying, et al. Responses of soil extracellular enzyme activities and microbial community properties to interaction between nitrogen addition and increased precipitation in a semi-arid grassland ecosystem [J/OL]. Science of the Total Environment, 2020, 703: 134691[2022-09-20]. doi: 10.1016/j.scitotenv.2019.134691. [72] WANG Ruzhen, CAO Yanzhuo, WANG Hongyi, et al. Exogenous P compounds differentially interacted with N availability to regulate enzymatic activities in a meadow steppe [J]. European Journal of Soil Science, 2020, 71(4): 667 − 680. [73] CHEN Hao, LI Dejun, ZHAO Jie, et al. Nitrogen addition aggravates microbial carbon limitation: evidence from ecoenzymatic stoichiometry [J]. Geoderma, 2018, 329: 61 − 64. [74] XU Hongwei, QU Qing, LI Guanwen, et al. Impact of nitrogen addition on plant-soil-enzyme C-N-P stoichiometry and microbial nutrient limitation [J/OL]. Soil Biology and Biochemistry, 2022, 170: 108714[2022-09-20]. doi: 10.1016/J.SOILBIO.2022.108714. [75] YUAN Xiaobo, NIU Decao, GHERARDI L A, et al. Linkages of stoichiometric imbalances to soil microbial respiration with increasing nitrogen addition: evidence from a long-term grassland experiment [J/OL]. Soil Biology and Biochemistry, 2019, 138: 107580[2022-09-20]. doi: 10.1016/j.soilbio.2019.107580. [76] LIU Meihua, GAN Bingping, LI Quan, et al. Effects of nitrogen and phosphorus addition on soil extracellular enzyme activity and stoichiometry in Chinese Fir (Cunninghamia lanceolata) forests [J/OL]. Frontiers in Plant Science, 2022, 13: 834184[2022-09-20]. doi: 10.3389/FPLS.2022.834184. [77] TATARIW C, MACRAE J D, FERNANDEZ I J, et al. Chronic nitrogen enrichment at the watershed scale does not enhance microbial phosphorus limitation [J]. Ecosystems, 2018, 21(1): 178 − 189. [78] GOMEZ E J, DELGADO J A, GONZALEZ J M. Persistence of microbial extracellular enzymes in soils under different temperatures and water availabilities [J]. Ecology and Evolution, 2020, 10(18): 10167 − 10176. [79] SHAW A N, CLEVELAND C C. The effects of temperature on soil phosphorus availability and phosphatase enzyme activities: a cross-ecosystem study from the tropics to the Arctic [J]. Biogeochemistry, 2020, 151(2): 113 − 125. [80] ZHOU Xiaoqi, CHEN Chengrong, WANG Yanfen, et al. Warming and increased precipitation have differential effects on soil extracellular enzyme activities in a temperate grassland [J]. Science of the Total Environment, 2013, 444: 552 − 558. [81] 刘珊杉, 周文君, 况露辉, 等. 亚热带常绿阔叶林土壤胞外酶活性对碳输入变化及增温的响应[J]. 植物生态学报, 2020, 44(12): 1262 − 1272. LIU Shanshan, ZHOU Wenjun, KUANG Luhui, et al. Responses of soil extracellular enzyme activities to carbon input alteration and warming in a subtropical evergreen broad-leaved forest [J]. Chinese Journal of Plant Ecology, 2020, 44(12): 1262 − 1272. [82] KRISHNAN A, CONVEY P, GONZALEZ M, et al. Effects of temperature on extracellular hydrolase enzymes from soil microfungi [J]. Polar Biology, 2018, 41(3): 537 − 551. [83] MENG Cheng, TIAN Dashuan, ZENG Hui, et al. Global meta-analysis on the responses of soil extracellular enzyme activities to warming [J/OL]. Science of the Total Environment, 2020, 705: 135992[2022-09-20]. doi: 10.1016/j.scitotenv.2019.135992. [84] CHEN Xiao, FENG Jiguang, DING Zongju, et al. Changes in soil total, microbial and enzymatic C-N-P contents and stoichiometry with depth and latitude in forest ecosystems [J/OL]. Science of the Total Environment, 2022, 816: 151583[2022-09-20]. doi: 10.1016/J.SCITOTENV.2021.151583. [85] OLAGOKE F K, KAISER K, MIKUTTA R, et al. Persistent activities of extracellular enzymes adsorbed to soil minerals [J/OL]. Microorganisms, 2020, 8(11): 1796[2022-09-20]. doi: 10.3390/microorganisms8111796. [86] CHEN Ji, LUO Yiqi, GARCIA-PALACIOS P, et al. Differential responses of carbon-degrading enzyme activities to warming: Implications for soil respiration [J]. Global Change Biology, 2018, 24(10): 4816 − 4826. [87] ZHENG Haifeng, LIU Yang, CHEN Yamei, et al. Short-term warming shifts microbial nutrient limitation without changing the bacterial community structure in an alpine timberline of the eastern Tibetan Plateau [J/OL]. Geoderma, 2020, 360: 113985[2022-09-20]. doi: 10.1016/j.geoderma.2019.113985. [88] GUAN Pingting, YANG Jingjing, YANG Yurong, et al. Land conversion from cropland to grassland alleviates climate warming effects on nutrient limitation: Evidence from soil enzymatic activity and stoichiometry [J/OL]. Global Ecology and Conservation, 2020, 24: e01328[2022-10-20]. doi: 10.1016/j.gecco.2020.e01328. [89] WANG Qitong, CHEN Lanying, XU Hang, et al. The effects of warming on root exudation and associated soil N transformation depend on soil nutrient availability [J/OL]. Rhizosphere, 2021, 17: 100263[2022-09-20]. doi: 10.1016/j.rhisph.2020.100263. [90] FANG Xiong, ZHOU Guoyi, LI Yuelin, et al. Warming effects on biomass and composition of microbial communities and enzyme activities within soil aggregates in subtropical forest [J]. Biology and Fertility of Soils, 2016, 52(3): 353 − 365. [91] LI Huayong, TIAN Haixia, WANG Ziquan, et al. Potential effect of warming on soil microbial nutrient limitations as determined by enzymatic stoichiometry in the farmland from different climate zones [J/OL]. Science of the Total Environment, 2022, 802: 149657[2022-09-20]. doi: 10.1016/J.SCITOTENV.2021.149657. [92] LIE Zhiyang, LIN Wei, HUANG Wenjuan, et al. Warming changes soil N and P supplies in model tropical forests [J]. Biology and Fertility of Soils, 2019, 55(7): 751 − 763. [93] MONTIEL-GONZALEZ C, TAPIA-TORRES Y, SOUZA V, et al. The response of soil microbial communities to variation in annual precipitation depends on soil nutritional status in an oligotrophic desert [J/OL]. PeerJ, 2017, 5(11): e4007[2022-09-20]. doi: 10.7717/peerj.4007. [94] ZHANG Shuohong, PAN Ying, ZHOU Zhenghu, et al. Resource limitation and modeled microbial metabolism along an elevation gradient [J/OL]. Catena, 2022, 209: 105807[2022-09-20]. doi: 10.1016/J.CATENA.2021.105807. [95] SIMPSON R M, MASON K, ROBERTSON K, et al. Relationship between soil properties and enzyme activities with soil water repellency [J]. Soil Research, 2019, 57(6): 689 − 702. [96] LI Xingfu, ZHANG Ying, DING Chengxiang, et al. Water addition promotes vegetation recovery of degraded alpine meadows by regulating soil enzyme activity and nutrients in the Qinghai-Tibetan Plateau [J/OL]. Ecological Engineering, 2020, 158: 106047[2022-09-20]. doi: 10.1016/j.ecoleng.2020.106047. [97] AKINYEMI D S, ZHU Yankun, ZHAO Mengying, et al. Response of soil extracellular enzyme activity to experimental precipitation in a shrub-encroached grassland in Inner Mongolia [J/OL]. Global Ecology and Conservation, 2020, 23: e01175[2022-09-20]. doi: 10.1016/j.gecco.2020.e01175. [98] 刘雄, 罗超, 向元彬, 等. 模拟降水量变化对华西雨屏区天然常绿阔叶林土壤酶活性的影响[J]. 应用与环境生物学报, 2020, 26(3): 635 − 642. LIU Xiong, LUO Chao, XIANG Yuanbin, et al. Effects of simulated precipitation changes on soil enzyme activities in a natural, evergreen, broad-leaf forest in the rainy area of western China [J]. Chinese Journal of Applied and Environmental Biology, 2020, 26(3): 635 − 642. [99] 柴锦隆, 徐长林, 张德罡, 等. 模拟践踏和降水对高寒草甸土壤养分和酶活性的影响[J]. 生态学报, 2019, 39(1): 333 − 344. CHAI Jinlong, XU Changlin, ZHANG Degang, et al. Effects of simulated trampling and rainfall on soil nutrients and enzyme activity in an alpine meadow [J]. Acta Ecologica Sinica, 2019, 39(1): 333 − 344. [100] CUI Yongxing, FANG Linchuan, DENG Lei, et al. Patterns of soil microbial nutrient limitations and their roles in the variation of soil organic carbon across a precipitation gradient in an arid and semi-arid region [J]. Science of the Total Environment, 2019, 658: 1440 − 1451. [101] LI Jiwei, XIE Jiangbo, ZHANG Yu, et al. Interactive effects of nitrogen and water addition on soil microbial resource limitation in a temperate desert shrubland [J]. Plant and Soil, 2022, 475(1): 361 − 378. [102] LADWIG L M, SINSABAUGH R L, COLLINS S L, et al. Soil enzyme responses to varying rainfall regimes in Chihuahuan Desert soils [J]. Ecosphere, 2015, 6(3): 1 − 10. [103] LI Jiwei, DONG Lingbo, LIU Yulin, et al. Soil organic carbon variation determined by biogeographic patterns of microbial carbon and nutrient limitations across a 3, 000- km humidity gradient in China [J/OL]. Catena, 2022, 209(2): 13[2022-09-20]. doi: 10.1016/J.CATENA.2021.105849. [104] ROMERO-OLIVARES A L, ALLISON S D, TRESEDER K K. Soil microbes and their response to experimental warming over time: a meta-analysis of field studies [J]. Soil Biology and Biochemistry, 2017, 107: 32 − 40. [105] KEANE J B, HOOSBEEK M R, TAYLOR C R, et al. Soil C, N and P cycling enzyme responses to nutrient limitation under elevated CO2 [J]. Biogeochemistry, 2020, 151(2): 221 − 235. [106] FINZI A C, SINSABAUGH R L, LONG T M, et al. Microbial community responses to atmospheric carbon dioxide enrichment in a warm-temperate forest [J]. Ecosystems, 2006, 9(2): 215 − 226. [107] FANG Huajun, CHENG Shulan, LIN Erda, et al. Elevated atmospheric carbon dioxide concentration stimulates soil microbial activity and impacts water-extractable organic carbon in an agricultural soil [J]. Biogeochemistry, 2015, 122(2): 253 − 267. [108] STEINWEG J M, DUKES J S, PAUL E A, et al. Microbial responses to multi-factor climate change: effects on soil enzymes [J/OL]. Frontiers in Microbiology, 2013, 4: 146[2022-09-20]. doi: 10.3389/fmicb.2013.00146. [109] PHILLIPS R P, FINZI A C, BERNHARDT E S. Enhanced root exudation induces microbial feedbacks to N cycling in a pine forest under long-term CO2 fumigation [J]. Ecology Letters, 2011, 14(2): 187 − 194. [110] OCHOA-HUESO R, HUGHES J, DELGADO-BAQUERIZO M, et al. Rhizosphere-driven increase in nitrogen and phosphorus availability under elevated atmospheric CO2 in a mature Eucalyptus woodland [J]. Plant and Soil, 2017, 416(1/2): 283 − 295. [111] MEIER I C, PRITCHARD S G, BRZOSTEK E R, et al. The rhizosphere and hyphosphere differ in their impacts on carbon and nitrogen cycling in forests exposed to elevated CO2 [J]. New Phytologist, 2015, 205(3): 1164 − 1174. [112] DORODNIKOV M, BLAGODATSKAYA E, BLAGODATSKY S, et al. Stimulation of microbial extracellular enzyme activities by elevated CO2 depends on soil aggregate size [J]. Global Change Biology, 2009, 15(6): 1603 − 1614. -
-
链接本文:
https://zlxb.zafu.edu.cn/article/doi/10.11833/j.issn.2095-0756.20220619
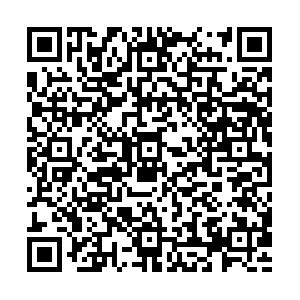
计量
- 文章访问数: 1001
- HTML全文浏览量: 75
- PDF下载量: 107
- 被引次数: 0