-
植物叶片含有叶绿素、叶黄素、花青素、类胡萝卜素等多种色素,正常生育期的叶片中叶绿素占据绝对优势而使得叶片呈现绿色[1]。叶片颜色变异是高等植物中一种普遍且易于识别的性状变异。由于自然突变或人工诱变,植物体内色素合成代谢相关基因发生变化,直接或间接地影响包括叶绿素在内的多种色素的合成或降解,使得叶色突变体中这些色素的质量分数和比例发生变化,植物叶片随之呈现不同的颜色[2]。通常叶色突变体的叶绿体结构会发生改变,叶绿体发生形变、外膜破损等,使得叶绿体无法维持正常功能,叶绿素的合成减少,光合作用减弱,导致植物生长发育不良甚至死亡[3]。因此,在很长一段时间内叶色变异被认为是没有价值的有害变异[4],直至有研究利用普通小球藻绿色突变体W5证明原卟啉Ⅲ是叶绿素合成的前体后[5],叶色突变体又得到重视,作为优良的实验材料在叶色调控机制、色素合成代谢途径、叶绿体结构、光合作用机制等基础研究中发挥着重要作用。另外,调控叶色的基因可作为选择标记基因应用在分子育种和杂交育种中;具有观赏性的叶色突变体也是优秀的彩叶植物种质资源,可被应用于园林绿化中。本文对近些年叶色突变体在来源、叶色性状分类、光合特性、叶色变异分子机制、应用价值等方面的研究进行综述,以期对叶色突变体研究提供依据和新的思路。
-
高等植物的叶色突变体主要来源于自然突变和人工诱变。相较于人工诱变而言,自然突变的数量较少,人工诱变是植物叶色突变体的主要来源方式,人工诱变又可分为化学试剂诱导[6]、物理射线诱导[7]和转基因诱导[8]等。
叶色变异普遍在苗期就表现出不同的叶色变化,因此一般以苗期的叶色为依据对叶色突变体进行分类。GUSTAFSSON[9]将叶色突变体分为白化、浅绿、黄化、条纹和斑点等5种类型。而后,AWAN等[10]进行了更加详细的分类,将叶色突变体分为黄化、黄绿、绿黄、绿白、白化、浅绿、白翠和条纹。WALLES[11]则根据突变体的叶片在其整个生育期颜色的变化方式,分为三大类:单色突变、杂色突变、阶段性失绿。单色突变表现为仅出现黄、白或浅绿中的一种颜色;杂色突变表现为斑点、白绿等多种颜色;阶段性失绿则在生育期的某一阶段表现出突变性状,一段时间后又恢复为绿色,如大麦Hordeum vulgare突变体whs18在苗期表现为绿色,从第7叶或第8叶开始黄化,而后短时间出现白化叶,在拔节前长出的新叶呈现条纹白色,而后又逐渐长出正常的绿叶[12];常绿则是在生育末期仍保持绿色的性状且不易黄化,如在拟南芥Arabidopsis thaliana中由于编码脱镁叶绿素水解酶的基因PPH发生突变,使得叶绿素降解途径中脱镁叶绿素转化为脱镁叶绿素酸a过程受到阻碍而出现的常绿突变体[13]。
植物叶色突变体的遗传通常分为细胞核遗传、细胞质遗传和核质互作遗传等3种方式。大多数叶色突变体为细胞核遗传,如绿豆Vigna radiata叶绿素缺乏突变体[14]、黄瓜Cucumis sativus黄叶突变体[15]等都为细胞核遗传。叶绿体具有相对独立的基因表达和调控系统[16],部分叶色突变体表现为细胞质遗传,如玉米Zea mays条纹叶突变体[17]、小麦Triticum aestivum紫条纹叶色突变体psl1[18]等。叶绿体的发育代谢等过程需要核基因与叶绿体基因的协调作用,核基因突变会影响叶绿体基因的表达[19−20],因此,在少部分情况下叶色突变体表现为核质互作,如经2-4-氯苯巯基三乙胺(CPTA)处理得到的大麦叶色突变体[21]。
与正常绿色叶片植株相比,由于叶色突变体叶片色素比例的改变、叶绿体结构的形变及叶绿体数量的减少,突变体的光合作用受到抑制[22]。大多数叶色突变体叶片的净光合速率下降、二氧化碳利用率降低,从而使得叶片的细胞间二氧化碳摩尔分数增加,并且净光合速率和蒸腾速率都与气孔导度呈现正相关[23−24],如牡丹Paeonia suffruticosa黄叶突变体的光合参数明显低于野生型牡丹,相较于野生型,突变体的净光合速率、气孔导度、蒸腾速率都下降了50%以上,胞间二氧化碳摩尔分数则增加了15.1%[25]。
-
叶绿素的生物合成途径由一系列酶催化的合成步骤组成[26],反应以谷氨酸酰-tRNA为起始,在谷氨酰胺tRNA还原酶的催化下转化为谷氨酸-1-半醛,紧接着经过3步酶催化反应合成胆色素原。研究表明:催化合成胆色素原的胆色素原脱氨酶(PBGD)的缺陷会引起玉米的叶色变化,突变体中编码PBGD的基因发生突变,PBGD的催化活性降低,叶绿素合成量减少,最终形成浅绿色的斑纹叶片[27]。经过尿卟啉原Ⅲ合成酶、尿卟啉原Ⅲ脱羧酶、粪卟啉原Ⅲ氧化酶等酶催化的一系列酶促反应后,胆色素原转化为镁原卟啉Ⅸ单甲酯。镁原卟啉Ⅸ单甲酯在镁原卟啉单甲酯Ⅸ环化酶(MgPCY)的催化下转化为二乙烯原叶绿素酸酯,在原叶绿素酸酯还原酶(POR)和联乙烯还原酶(DVR)的催化下合成单乙烯叶绿素a。研究证明:编码MgPCY[28]、POR[29]、DVR[30−31]的基因发生突变,使得酶功能丧失,叶绿素合成受阻,植物叶片则表现出不同程度的失绿。单乙烯叶绿素a在叶绿素合酶的催化下合成叶绿素a,在叶绿素酸酯a加氧酶(CAO)和叶绿素b还原酶的催化下最终合成叶绿素b。在大白菜Brassica rapa ssp. pekinensis浅绿叶突变体中,编码CAO的基因发生单核苷酸突变,蛋白质翻译提前结束,CAO活性显著降低,叶绿素b合成量减少[32]。叶绿素生物合成途径中催化反应进行的酶,尤其是叶绿素生物合成途径中的关键酶受损后,植物体叶绿素的合成随之受到抑制。催化卟啉原Ⅸ转化为镁卟啉原Ⅸ的镁螯合酶是叶绿素生物合成的限速酶和关键酶,它由3个亚基组成,分别为镁螯合酶I亚基(CHLI)、镁螯合酶D亚基(CHLD)、镁螯合酶H亚基(CHLH)[33−34]。编码这3个亚基的基因发生突变的植物,镁螯合酶的正常功能受损,叶绿素的生物合成受阻,叶片都表现出黄色或黄绿色的性状[35−37]。镁螯合酶催化反应需要能量,CHLI具有ATP酶活性,能够水解ATP,促进反应的进行[38]。基因组解偶联4蛋白(GUN4)是一种核基因编码的蛋白,它与卟啉原Ⅸ结合形成复合物,再与CHLH-GUN5复合物结合,刺激镁螯合酶的活性,促进镁离子插入卟啉原Ⅸ形成镁卟啉原Ⅸ[39−40]。INAGAKI等[41]在水稻Oryza sativa中发现:GUN4的合成受到光敏色素B的转录调控,突变体中的GUN4基因受到强烈抑制,镁螯合酶活性减弱,水稻叶片呈现淡绿色。GUN4表现出光依赖性。在黑暗中,拟南芥中的GUN4受GUN4激酶调节,当其C端被磷酸化,GUN4失去活性,拟南芥叶片出现褪绿现象[42]。叶绿素生物合成途径中的任意一个合成步骤受到各种因素的影响而中断都会影响叶绿素的生物合成,随之植物体内的叶绿素质量分数降低,从而导致植物叶色的改变[43]。
-
在植物体内,叶绿素的合成和代谢是同步进行的,维持着动态平衡。当代谢过程中相关基因发生突变,这种动态平衡的改变会引起植物叶色的变化[44]。目前,已经有许多叶绿素分解代谢相关基因被发现,包括SGR1(也被称为NYE1)、SGR2(也被称为NYE2)、NYC1、NOL、脱镁叶绿酸a加氧酶基因(PAO)、脱镁叶绿酸a水解酶基因(PPH)等,并且这些基因受到不同的转录因子及转录调控机制的调控[45−52]。茉莉酸被认为是植物衰老的诱导剂,可调控叶绿素的降解,转录调控因子MYCs是茉莉酸诱导的叶绿素降解途径的关键调控节点[46−47]。MYCs可通过与PAO的启动子结合,直接促进叶绿素降解。同时MYCs可与转录调控因子ANACs结合,形成复合物,激活NYE1和NYC1的表达,从而促进叶绿素的降解。ANACs也可直接激活叶绿素代谢相关基因,促使叶绿素降解[46−48]。PIF4/5是一种光敏色素作用因子,在光信号转导中的起着重要的调控作用,对叶绿素降解具有正向调控作用[49]。PIF4/5可直接调控叶绿素代谢相关基因,如SGR、NYC1、NOL等,促进叶绿素降解[50]。另外,在黑暗及高温条件下,PIF4/5能够正向调控转录因子ORE1[50]。ORE1在衰老过程中激活乙烯生物合成基因ACS2的表达,随后激活乙烯介导的叶绿素降解网络[51]。乙烯不敏感3(EIN3)是乙烯信号传导的主要正调节因子,可以与NYE1、NYC1、PAO的启动子物理结合以激活其表达来直接促进叶绿素降解,同时EIN3也可直接靶向ORE1,从而激活叶绿素降解相关基因的表达[51]。植物叶绿素代谢过程中的基因上调或下调都会影响叶绿素的代谢过程,使得植物叶片出现失绿或常绿的现象[53]。
-
类胡萝卜素是使植物呈现黄色、橙色或红色的一类色素。根据化学结构的不同可以将类胡萝卜素分为2类:一类是胡萝卜素,如 α-胡萝卜素、β-胡萝卜素和番茄红素;另一类是叶黄素,如玉米黄素、叶黄素和虾青素[54]。类胡萝卜素的生物合成由香叶基香叶基焦磷酸酯(GGPP)开始,经过一系列的酶促反应后合成上述2类类胡萝卜素。八氢番茄红素合成酶(PSY)催化类胡萝卜素生物合成的第1步反应,而后八氢番茄红素在八氢番茄红素脱氢酶(PDS)的催化下转化为顺式番茄红素。研究表明:PSY基因和PDS基因缺陷的植物,其类胡萝卜素生物合成途径受阻,同时影响叶绿素生物合成途径,植物叶片出现白化现象[55−56]。合成途径分支处的类胡萝卜素异构酶(CRTISO)是类胡萝卜素生物合成中的关键酶,它催化顺式番茄红素转化为全反式番茄红素[57]。SU等[58]和LI等[59]研究发现:大白菜突变体中的类胡萝卜素异构酶基因BrCRTISO存在不同程度的片段缺失以及单核苷酸突变,类胡萝卜素异构酶的功能缺失导致顺式番茄红素大量积累,然而这一步反应可在光诱导下进行非酶反应,因此突变体的外叶呈现绿色,因没有光照无法继续合成叶黄素、玉米黄素等类胡萝卜素,顺式番茄红素大量积累,故内叶呈现橙色。类胡萝卜素叶绿体调节剂1基因CCR1编码组蛋白甲基转移酶(SET结构域8,SDG8),SDG8能与CRTISO的启动子结合,调控类胡萝卜素的生物合成[60−61]。
-
类黄酮是在植物中普遍存在的色素,通常为浅黄色或无色,偶尔表现为橙黄色,同时也是一种重要次生代谢物,大多数类黄酮具有显著的生理及药理活性[62]。迄今为止,从植物中分离得到的黄酮类化合物有9 000多种[63]。类黄酮生物合成途径非常复杂,通过苯丙氨酸途径将苯丙氨酸转化为各种黄酮类化合物[64]。苯丙氨酸氨裂解酶(PAL)、查尔酮合成酶(CHS)、查尔酮还原酶(CHR)、查尔酮异构酶(CHI)等催化类黄酮生物合成的酶的变化会使得植物叶片中黄酮类化合物的质量分数发生变化,导致叶片颜色的变化[65−66]。花青素是一类广泛存在于植物中的水溶性类黄酮色素,在不同的pH下会使植物呈现不同的颜色,并且对植物抗氧化应激具有重要的调节作用[67]。类黄酮生物合成途径分支处的柚皮苷查尔酮在CHI、黄酮3’ 羟化酶(F3’ H)、二氢黄酮醇4还原酶(DFR)以及花青素还原酶(ANS)的催化下合成花青素。有研究比较了枫香Liquidambar formosana转色期的基因表达量,发现花青素合成途径中的CHS、CHI、F3’ H、DFR、ANS等酶基因的表达量显著增加[68]。结构基因的转录调控是植物中调节花青素生物合成的重要机制,在紫叶茶Camellia sinensis[69]、紫叶芥菜Brassica juncea[70]、紫叶大琴叶榕Ficus lyrate[71]和具有斑纹叶片的蒺藜苜蓿Medicago truncatula[72]中都发现了这种调节机制,即MYB转录因子与bHLH转录因子结合并招募WD40重复蛋白,形成MYB-bHLH-WD40复合物,调节花青素的合成,MYB转录因子的过度表达使得花青素的合成增加,出现紫色叶片。在蒺藜苜蓿中,拮抗性的MYB转录因子与促进花青素生物合成的MYB转录因子竞争性结合bHLH转录因子,导致形成斑纹叶片[72]。
-
叶绿体是植物所特有的“能量转换器”,植物的光合作用在此进行,将光能转换为植物体可利用的化学能,在植物的生长发育过程中发挥着重要的作用[73]。许多研究表明:叶色的变化往往伴随着叶绿体结构的改变,可表现为叶绿体液泡化[74],叶绿体数量减少,并伴随着不同程度的形变[75],其外膜也出现不同程度的破损[76],叶绿体内基粒的数量及基粒片层数量减少,基粒排列不整齐,变得松散[77−78]。叶绿体结构的变化及叶绿体的缺失可能会影响叶绿素等光合色素的质量分数和比例,这是导致植物叶色变化的重要原因之一[79−80]。
叶绿体蛋白由核基因与叶绿体基因编码合成,是叶绿体膜的重要组成成分,参与叶绿体的分化与发育。类囊体是组成叶绿体内膜系统的单位,类囊体膜上含有多种蛋白质,包括细胞色素b6/f复合体、铁氧化还原蛋白、黄素蛋白、光系统Ⅰ复合物、光系统Ⅱ复合物等[81]。Snowy Cotyledon 2(SCO2)蛋白是一类参与类囊体膜生物合成,并与光捕获叶绿素结合蛋白LHCB1相互作用的蛋白[82]。SCO2基因的缺失影响了叶绿体蛋白的合成,导致类囊体膜上的PSⅡ蛋白复合体的组装和修复受阻,从而导致百脉根Lotus japonicus和拟南芥子叶白化,真叶出现杂色[82]。五肽重复序列(PPR)蛋白是一类参与细胞器基因表达的转录后调控的蛋白质,在叶绿体发育中起着重要作用[83−84]。在拟南芥[85−86]和水稻[87−88]中都发现了多种PPR蛋白,并且水稻编码PPR蛋白的基因缺失突变体表现出白化叶片的表型[89−90]。多器官RNA编辑因子(Morf)是另一类参与转录后调控的蛋白,它可以与PPR蛋白形成复合物参与调节叶绿体基因的表达[91−92]。如在水稻中OsMORF9可以与五肽重复家族成员OsSLA4和DUA1相互作用,参与RNA剪接和编辑过程,影响叶绿体的发育[93]。
-
草本植物大多为1年生或2年生,其茎部木质化程度较低。草本植物茎部含有叶绿体,能进行光合作用[94],在叶色变异时往往会伴随着茎部颜色的变化。木本植物为多年生,茎部木质化程度高,具有保护作用的周皮由死亡的细胞组成[94]。木本植物叶色变异时,在其茎秆上往往观察不到颜色的变化。从根本上来说,草本植物与木本植物叶色变异都是由于色素合成代谢相关基因的变异,加上环境因素,直接或间接地影响色素的合成代谢,使得叶片中各种色素的质量分数及比例改变,最终形成不同的叶色[95]。
-
近些年,叶色突变体是遗传模式[96]、色素合成[97]、光合作用[98]、细胞结构与发育等基础研究的理想材料,并成为分子生物学和发育生物学的热点研究领域之一[99]。如从籼稻品种‘TN1’的甲磺酸乙酯诱变群体中分离出的稳定遗传的白色类病变突变体,其叶绿体超微结构发生改变,叶绿素代谢和叶绿体发育相关基因的调控发生改变,可作为研究叶绿体超微结构、叶绿素合成代谢、叶绿体发育和遗传模式的优良材料[100]。目前,水稻、拟南芥等模式植物叶色突变体的研究比较深入,有许多涉及叶绿素生物合成的基因已被克隆,如水稻中编码叶绿素生物合成途径中的关键酶——镁鳌合酶的ChlD亚基的同位基因YGL[101]、Chl1[102]、YGL7[103]等。
-
叶色常在苗期表达,并且极易识别,在育种中可以简化良种繁育[104]和杂交育种[105]过程,缩短育种年限,促进育种进程和提高种质资源利用效率。叶色变化可被肉眼轻易观察到,与叶色相关的基因可作为分子标记应用于选择标记系统。将目的基因和相关叶色基因同时调控,可实现通过观察叶片颜色筛选出表达目的基因的转基因植株[106]。核编码的δ因子对于植物叶绿体编码基因的转录调控至关重要。在玉米中,由于δ因子ZmSig2A的缬氨酸残基缺失导致形成可逆黄化/白化叶突变体[107],利用该突变基因进行杂交育种时,可在苗期时就识别和消除假杂种。敲除植物体内PDS基因会引起叶片白化,所以常被用于检测基因编辑体系是否成功[108]。近年来,利用PDS基因与成簇的规律间隔的短回文重复序列(CRISPR/Cas9)基因编辑技术对植物进行基因编辑,在植物遗传改良及种质创新等方面广泛应用[109]。
一些叶色突变体在产量、风味、观赏等方面具有优异表现,许多叶色突变体被认为是优良种质资源。可利用一些作物叶片常绿的性状延缓衰老、增加作物的产量,如水稻的叶绿素代谢途径受阻,叶片保持常绿,种子中的多种营养物质含量增加[110];或因叶片颜色变化使得代谢物含量不同,从而具有独特的风味,如茶树Camellia sinensis白化品种‘玉金香’叶色金黄,叶片中槲皮素和儿茶素质量分数增加使其更具有茶味[111]。一些彩叶植物颜色绚丽、枝叶繁茂,作为观赏植物在园林绿化中得到广泛应用,常见的有金叶女贞Ligustrum × vicaryi、金边黄杨Euonymus japonicus ‘Aurea-marginatus’、枫香、银杏Ginkgo biloba等[112]。
-
由于叶色突变体大多表现为失绿,影响植物体的光合作用,进而影响植物的生长发育,因此在最初被认为是不良性状,对于叶色突变体的研究较少。直到发现叶色突变体是研究色素合成、叶绿体结构、光合作用等的优良材料,对叶色突变体的研究才逐渐增多。叶色变异在植物中非常广泛,虽然有关叶色突变体的研究已较多,但是大多集中在模式植物拟南芥以及水稻、玉米等经济作物上,并且大多利用转录组、蛋白质组、代谢组等大数据联合分析的方法对叶绿素生物合成代谢途径上的基因进行研究,而对叶色变异的关键基因的上游调控机制的研究较少,如一些具有调控作用的非编码RNA在叶色变异中的作用。因此,后续的研究应利用更丰富的分子生物学手段,从多方面进一步解析叶色变异的机制,为更多植物叶色突变体的研究提供理论依据。
Research progress on classification, variation mechanism and application value of plant leaf color mutants
-
摘要: 植物叶色变异是自然界中一种常见并且容易识别的现象,叶色变异通常会影响植物的光合作用效率,导致生长不良,因此曾被认为是不良变异而逐渐被淘汰。但近年来,随着社会的发展和园林绿化的需求,叶色突变体逐渐受到关注。叶色突变体受到分子水平的调控,导致叶绿体结构异常,光合作用受抑制,色素合成代谢通路异常,因此是研究色素代谢、叶绿体发育分化、光合作用等途径的理想材料,也可为品种改良提供重要信息。本文综述了近年来植物叶色突变体的研究进展,明确高等植物叶色突变体主要来源于自然突变和人工诱变,叶色突变体按颜色可分为黄化、黄绿、绿黄、绿白、白化、浅绿、白翠、条纹等几种类型;按叶色变化方式可分为单色突变、杂色突变和阶段性失绿,其遗传方式通常分为细胞核遗传、细胞质遗传和核质互作遗传;与正常绿色叶片植株相比,大多数叶色突变体叶片的净光合速率、气孔导度和蒸腾速率都下降,二氧化碳的利用率降低;叶绿素、类胡萝卜素、类黄酮(主要是花青素)合成与代谢相关基因的突变以及与叶绿体分化发育相关基因的突变可解释叶色突变体发生的分子机制。叶色突变体可应用于园林绿化,并作为优良的实验材料广泛应用在基础研究和育种工作。 参112Abstract: Leaf color variation in plants is a common and easily recognized phenomenon in nature, which affects the photosynthetic efficiency of plants and leads to poor growth, so it was once considered to be an undesirable variation and gradually eliminated. But in recent years, social advancements and increased demand for ornamental landscaping have brought renewed attention to leaf color mutants. Leaf color mutants are generally regulated at the molecular level, resulting in abnormal chloroplast structure, inhibited photosynthesis, and abnormal pigment synthesis and metabolic pathways. Therefore, they serve as ideal materials for studying pigment metabolism, chloroplast development and differentiation, photosynthesis, and related pathways, and can also provide important information for variety improvement. This paper reviews the research progress of leaf color mutants in recent years, and clarifies that leaf color mutants in higher plants mainly come from natural mutation and artificial mutation. Leaf color mutants can be divided into several types based on color, such as yellowing, yellow green, green yellow, green white, albino, light green, white emerald, stripe and so on. According to the way of leaf color change, leaf color mutants can be divided into monochromatic mutation, variegated mutation and stage chlorosis. Its inheritance mode is generally divided into nuclear inheritance, cytoplasmic inheritance and nucleocytoplasmic interaction inheritance. Compared with normal green leaf plants, the net photosynthetic rate, stomatal conductance, transpiration rate of most leaf color mutants decrease, so does the utilization rate of carbon dioxide. Mutations in genes related to synthesis and metabolism of chlorophyll, carotenoids, and flavonoids (particularly anthocyanins), as well as genes involved in chloroplast differentiation and development, can explain the molecular mechanism underlying leaf color mutants. Leaf color mutants can be applied in landscaping and widely used in basic research and breeding as excellent experimental materials. The findings of this review can provide theoretical reference for further research on leaf color variation. [Ch, 112 ref.]
-
Key words:
- leaf color variation /
- chlorophyll /
- chloroplast /
- carotenoid /
- flavonoid /
- review
-
[1] BOREK M, BACZEK-KWINTA R, RAPACZ M. Photosynthetic activity of variegated leaves of Coleus × hybridus hort. cultivars characterised by chlorophyll fluorescence techniques [J]. Photosynthetica, 2016, 54(3): 331−339. [2] LI Weixing, YANG Shunbo, LU Zhaogeng, et al. Cytological, physiological, and transcriptomic analyses of golden leaf coloration in Ginkgo biloba L [J/OL]. Horticulture Research, 2018, 5 : 32[2024-05-09]. DOI: 10.1038/s41438-018-0039-9. [3] XU Binhua, ZHANG Chaoyang, GU Yan, et al. Physiological and transcriptomic analysis of a yellow leaf mutant in watermelon [J/OL]. Scientific Reports, 2023, 13 (1): 9647[2024-05-09]. DOI: 10.1038/s41598-023-36656-6. [4] BEALE S I, APPLEMAN D. Chlorophyll synthesis in Chlorella: regulation by degree of light limitation of growth [J]. Plant Physiology, 1971, 47(2): 230−235. [5] GRANICK S. Protoporphyrin Ⅸ as a precursor of chlorophyll [J]. Journal of Biological Chemistry, 1948, 172(2): 717−727. [6] CAO Zhe, DENG Zhanao. Morphological, cytological and molecular marker analyses of ‘Tapestry’ caladium variants reveal diverse genetic changes and enable association of leaf coloration pattern loci with molecular markers [J]. Plant Cell, Tissue and Organ Culture (PCTOC), 2020, 143(2): 363−375. [7] ZHAO Shaolu, LONG Wuhua, WANG Yihua, et al. A rice white-stripe leaf3 (wsl3) mutant lacking an HD domain-containing protein affects chlorophyll biosynthesis and chloroplast development [J]. Journal of Plant Biology, 2016, 59(3): 282−292. [8] PARK S Y, YU J W, PARK J S, et al. The senescence-induced staygreen protein regulates chlorophyll degradation [J]. The Plant Cell, 2007, 19(5): 1649−1664. [9] GUSTAFSSON Å. The plastid development in various types of chlorophyll mutations [J]. Hereditas, 2010, 28(3/4): 483−492. [10] AWAN M A, KONZAK C F, RUTGER J N, et al. Mutagenic effects of sodium azide in rice [J]. Crop Science, 1980, 20(5): 663−668. [11] WALLES B. The homozygous and heterozygous effects of an aurea mutation on plastid development in spruce (Picea abies L. ) [J]. Studia Forestalia Suecica, 1967, 60: 1−20. [12] QIN Dandan, DONG Jing, XU Fuchao et al. Characterization and fine mapping of a novel barley stage green-revertible albino gene (HvSGRA) by bulked segregant analysis based on SSR assay and specific length amplified fragment sequencing [J/OL]. BMC Genomics, 2015, 16 : 838[2024-05-09]. DOI: 10.1186/s12864-015-2015-1. [13] SCHELBERT S, AUBRY S, BURLA B, et al. Pheophytin pheophorbide hydrolase (Pheophytinase) is involved in chlorophyll breakdown during leaf senescence in Arabidopsis [J]. The Plant Cell, 2009, 21(3): 767−785. [14] RUNGNOI O, CHANPREM S, TOOJINDA T, et al. Characterization, inheritance, and molecular study of opaque leaf mutant in mungbean (Vigna radiata (L. ) Wilczek) [J]. Journal of Crop Science and Biotechnology, 2010, 13(4): 219−226. [15] ZHA Gaohui, YIN Juan, CHENG Feng, et al. Fine mapping of CscpFtsY, a gene conferring the yellow leaf phenotype in cucumber (Cucumis sativus L. ) [J/OL]. BMC Plant Biology, 2022, 22 (1): 570[2024-05-09]. DOI: 10.1186/s12870-022-03922-0. [16] PFALZ J, PFANNSCHMIDT T. Essential nucleoid proteins in early chloroplast development [J]. Trends in Plant Science, 2013, 18(4): 186−194. [17] LIN B Y, YU H J. Inheritance of a striped-leaf mutant is associated with the cytoplasmic genome in maize [J]. Theoretical and Applied Genetics, 1995, 91: 915−920. [18] LIU Cong, SHI Narong, WU Huiyu, et al. Cytogenetic analyses of PSL1 mutant, a novel low-temperature-sensitive purple-striped leaf color mutant in wheat [J]. Crop Science, 2018, 58(5): 1919−1931. [19] HERNÁNDEZ-VERDEJA T, VUORIJOKI L, STRAND Å. Emerging from the darkness: interplay between light and plastid signaling during chloroplast biogenesis [J]. Physiologia Plantarum, 2020, 169(3): 397−406. [20] SHIMIZU T, KACPRZAK S M, MOCHIZUKI N, et al. The retrograde signaling protein GUN1 regulates tetrapyrrole biosynthesis [J]. Proceedings of the National Academy of Sciences of the United States of America, 2019, 116(49): 24900−24906. [21] ROCCA N L, RASCIO N, OSTER U, et al. Inhibition of lycopene cyclase results in accumulation of chlorophyll precursors [J]. Planta, 2007, 225(4): 1019−1029. [22] LIGUORI N, CROCE R, MARRINK S J, et al. Molecular dynamics simulations in photosynthesis [J]. Photosynthesis Research, 2020, 144(2): 273−295. [23] WANG Fenfen, CHEN Naizhi, SHEN Shihua. iTRAQ-based quantitative proteomics analysis reveals the mechanism of golden-yellow leaf mutant in hybrid paper mulberry [J/OL]. International Journal of Molecular Sciences, 2021, 23 (1): 127[2024-05-09]. DOI: 10.3390/ijms23010127. [24] GANG Huixin, LIU Guifeng, CHEN Su, et al. Physiological and transcriptome analysis of a yellow-green leaf mutant in birch (Betula platyphylla × B. pendula) [J/OL]. Forests, 2019, 10 (2): 120[2024-05-09]. DOI: 10.3390/f10020120. [25] CHANG Q S, ZHANG L X, HOU X G, et al. The anatomical, physiological, and molecular analysis of a chlorophyll-deficient mutant in tree peony (Paeonia suffruticosa) [J]. Photosynthetica, 2019, 57(3): 724−730. [26] MASUDA T, FUJITA Y. Regulation and evolution of chlorophyll metabolism [J]. Photochemical & Photobiological Sciences, 2008, 7(10): 1131−1149. [27] HUANG Mingshu, SLEWINSKI T L, BRAKER R F, et al. Camouflage patterning in maize leaves results from a defect in porphobilinogen deaminase [J]. Molecular Plant, 2009, 2(4): 773−789. [28] HUNG C Y, SUN Y H, CHEN Jianjun, et al. Identification of a Mg-protoporphyrin Ⅸ monomethyl ester cyclase homologue, EaZIP, differentially expressed in variegated Epipremnum aureum ‘Golden Pothos’ is achieved through a unique method of comparative study using tissue regenerated plants [J]. Journal of Experimental Botany, 2010, 61(5): 1483−1493. [29] KANG Shujing, FANG Yunxia, ZOU Guoxing, et al. White-green leaf gene encoding protochlorophyllide oxidoreductase B is involved in chlorophyll synthesis of rice [J]. Crop Science, 2015, 55(1): 284−293. [30] ZHAO Yonghui, HUANG Shengnan, ZHANG Meidi, et al. Mapping of a pale green mutant gene and its functional verification by allelic mutations in Chinese cabbage (Brassica rapa L. ssp. pekinensis) [J/OL]. Frontiers in Plant Science, 2021, 12 : 699308[2024-05-09]. DOI: 10.3389/fpls.2021.699308. [31] LONG Wuhua, LONG Sifang, JIANG Xue, et al. A rice Yellow-Green-Leaf 219 mutant lacking the divinyl reductase affects chlorophyll biosynthesis and chloroplast development [J]. Journal of Plant Growth Regulation, 2022, 41: 3233−3242. [32] ZHAO Yonghui, HUANG Shengnan, WANG Nan, et al. Identification of a biomass unaffected pale green mutant gene in Chinese cabbage (Brassica rapa L. ssp. pekinensis) [J/OL]. Scientific Reports, 2022, 12 : 7731[2024-05-09]. DOI: 10.1038/s41598-022-11825-1. [33] LU Wei, TENG Yantong, HE Fushou, et al. OsChlC1, a novel gene encoding magnesium-chelating enzyme, affects the content of chlorophyll in rice [J/OL]. Agronomy, 2023, 13 (1): 129[2024-05-09]. DOI: 10.3390/agronomy13010129. [34] HANSSON A, WILLOWS R D, ROBERTS T H, et al. Three semidominant barley mutants with single amino acid substitutions in the smallest magnesium chelatase subunit form defective AAA+ hexamers [J]. Proceedings of the National Academy of Sciences of the United States of America, 2002, 99(21): 13944−13949. [35] MA Yangyang, SHI Jiancheng, WANG Danjuan, et al. A point mutation in the gene encoding magnesium chelatase I subunit influences strawberry leaf color and metabolism [J]. Plant Physiology, 2023, 192(4): 2737−2755. [36] JUNG K H, HUR J, RYU C H, et al. Characterization of a rice chlorophyll-deficient mutant using the T-DNA gene-trap system [J]. Plant & Cell Physiology, 2003, 44(5): 463−472. [37] FU Wei, YE Xueling, REN Jie, et al. Fine mapping of lcm1, a gene conferring chlorophyll-deficient golden leaf in Chinese cabbage (Brassica rapa ssp. pekinensis) [J/OL]. Molecular Breeding, 2019, 39 (4): 52[2024-05-09]. DOI: 10.1007/s11032-019-0945-z. [38] JENSEN P E, REID J D, HUNTER C N. Modification of cysteine residues in the Chl I and Chl H subunits of magnesium chelatase results in enzyme inactivation [J]. The Biochemical Journal, 2000, 352(2): 435−441. [39] LARKIN R M, ALONSO J M, ECKER J R, et al. GUN4, a regulator of chlorophyll synthesis and intracellular signaling [J]. Science, 2003, 299(5608): 902−906. [40] ADHIKARI N D, FROEHLICH J E, STRAND D D, et al. GUN4-porphyrin complexes bind the ChlH/GUN5 subunit of Mg-Chelatase and promote chlorophyll biosynthesis in Arabidopsis [J]. The Plant Cell, 2011, 23(4): 1449−1467. [41] INAGAKI N, KINOSHITA K, KAGAWA T, et al. Phytochrome B mediates the regulation of chlorophyll biosynthesis through transcriptional regulation of ChlH and GUN4 in rice seedlings [J/OL]. PLoS One, 2015, 10 (8): e0135408[2024-05-09]. DOI: 10.1371/journal.pone.0135408. [42] RICHTER A S, HOCHHEUSER C, FUFEZAN C, et al. Phosphorylation of GENOMES UNCOUPLED 4 alters stimulation of Mg chelatase activity in Angiosperms [J]. Plant Physiology, 2016, 172(3): 1578−1595. [43] BEALE S I. Green genes gleaned [J]. Trends in Plant Science, 2005, 10(7): 309−312. [44] GRIMM B, PORRA R J, RÜDIGER W, et al. Chlorophylls and Bacteriochlorophylls [M]. Dordrecht: Springer Netherlands, 2006: 237−260. [45] SAKURABA Y, HAN S H, LEE S H, et al. Arabidopsis NAC016 promotes chlorophyll breakdown by directly upregulating STAYGREEN1 transcription [J]. Plant Cell Reports, 2016, 35 (1): 155−166. [46] CHEN Junyi, ZHU Xiaoyu, REN Jun, et al. Suppressor of overexpression of CO 1 negatively regulates dark-induced leaf degreening and senescence by directly repressing pheophytinase and other senescence-associated genes in Arabidopsis [J]. Plant Physiology, 2017, 173(3): 1881−1891. [47] ZHU Xiaoyu, CHEN Junyi, XIE Zuokun, et al. Jasmonic acid promotes degreening via MYC2/3/4- and ANAC019/055/072-mediated regulation of major chlorophyll catabolic genes [J]. The Plant Journal, 2015, 84(3): 597−610. [48] ODA-YAMAMIZO C, MITSUDA N, SAKAMOTO S, et al. The NAC transcription factor ANAC046 is a positive regulator of chlorophyll degradation and senescence in Arabidopsis leaves [J/OL]. Scientific Reports, 2016, 6: 23609 [2024-05-09]. DOI: 10.1038/srep23609. [49] ZHANG Yongqiang, LIU Zhongjuan, CHEN Yadi, et al. PHYTOCHROME-INTERACTING FACTOR 5 (PIF5) positively regulates dark-induced senescence and chlorophyll degradation in Arabidopsis [J]. Plant Science, 2015, 237: 57−68. [50] SONG Yi, YANG Chuangwei, GAO Shan, et al. Age-triggered and dark-induced leaf senescence require the bHLH transcription factors PIF3, 4, and 5 [J]. Molecular Plant, 2014, 7(12): 1776−1787. [51] QIU Kai, LI Zhongpeng, YANG Zhen, et al. EIN3 and ORE1 accelerate degreening during ethylene-mediated leaf senescence by directly activating chlorophyll catabolic genes in Arabidopsis [J/OL]. PLoS Genetics, 2015, 11 (7): e1005399[2024-05-09]. DOI: 10.1371/journal.pgen.1005399. [52] SAKURABA Y, PARK S Y, KIM Y S, et al. Arabidopsis STAY-GREEN2 is a negative regulator of chlorophyll degradation during leaf senescence [J]. Molecular Plant, 2014, 7(8): 1288−1302. [53] ZENG Zhaoqiong, LIN Tianzi, ZHAO Jieyu, et al. OsHemA gene, encoding glutamyl-tRNA reductase (GluTR) is essential for chlorophyll biosynthesis in rice (Oryza sativa) [J]. Journal of Integrative Agriculture, 2020, 19(3): 612−623. [54] JOHNSON J D. Do carotenoids serve as transmembrane radical channels [J]. Free Radical Biology and Medicine, 2009, 47(3): 321−323. [55] EZQUERRO M, BURBANO-ERAZO E, RODRIGUEZ-CONCEPCION M. Overlapping and specialized roles of tomato phytoene synthases in carotenoid and abscisic acid production [J]. Plant Physiology, 2023, 193(3): 2021−2036. [56] QIN Genji, GU Hongya, MA Ligeng, et al. Disruption of phytoene desaturase gene results in albino and dwarf phenotypes in Arabidopsis by impairing chlorophyll, carotenoid, and gibberellin biosynthesis [J]. Cell Research, 2007, 17(5): 471−482. [57] LU Shan, LI Li. Carotenoid metabolism: biosynthesis, regulation, and beyond [J]. Journal of Integrative Plant Biology, 2008, 50(7): 778−785. [58] SU Tongbing, YU Shuancang, ZHANG J W F, et al. Loss of function of the carotenoid isomerase gene BrCRTISO confers orange color to the inner leaves of Chinese cabbage (Brassica rapa L. ssp. pekinensis) [J]. Plant Molecular Biology Reporter, 2015, 33(3): 648−659. [59] LI Peirong, ZHANG Shujiang, ZHANG Shifan, et al. Carotenoid identification and molecular analysis of carotenoid isomerase-encoding BrCRTISO, the candidate gene for inner leaf orange coloration in Chinese cabbage [J/OL]. Molecular Breeding, 2015, 35 (2): 72[2024-05-09]. DOI: 10.1007/s11032-015-0190-z. [60] CAZZONELLI C I, CUTTRISS A J, COSSETTO S B, et al. Regulation of carotenoid composition and shoot branching in Arabidopsis by a chromatin modifying histone methyltransferase, SDG8 [J]. The Plant Cell, 2009, 21(1): 39−53. [61] CAZZONELLI C I, ROBERTS A C, CARMODY M E, et al. Transcriptional control of set domain group 8 and carotenoid isomerase during Arabidopsis development [J]. Molecular Plant, 2010, 3(1): 174−191. [62] DONG Naiqian, LIN Hongxuan. Contribution of phenylpropanoid metabolism to plant development and plant-environment interactions [J]. Journal of Integrative Plant Biology, 2021, 63(1): 180−209. [63] SUN Chenglong, ZHANG Minmin, DONG Hongjing, et al. A spatially-resolved approach to visualize the distribution and biosynthesis of flavones in Scutellaria baicalensis Georgi [J/OL]. Journal of Pharmaceutical and Biomedical Analysis, 2020, 179 : 113014[2025-05-09]. DOI: 10.1016/j.jpba.2019.113014. [64] LIU Weixin, FENG Yi, YU Suhang, et al. The flavonoid biosynthesis network in plants [J/OL]. International Journal of Molecular Sciences, 2021, 22 (23): 12824[2024-05-09]. DOI: 10.3390/ijms222312824. [65] LI Wenji, LI Huigen, SHI Lisha, et al. Leaf color formation mechanisms in Alternanthera bettzickiana elucidated by metabolite and transcriptome analyses [J/OL]. Planta, 2022, 255 (3): 59[2024-05-09]. DOI: 10.1007/s00425-022-03840-3. [66] HERRAIZ A, STOKES L, TURNBULL C, et al. Developing a new variety of Kentia palms (Howea forsteriana): up-regulation of cytochrome b561 and Chalcone synthase is associated with red colouration of the stems [J]. Botany Letters, 2018, 165(2): 241−247. [67] STEYN W J, WAND S E, HOLCROFT D M, et al. Anthocyanins in vegetative tissues: a proposed unified function in photoprotection [J]. The New Phytologist, 2002, 155(3): 349−361. [68] LI Yanjun, ZHOU Yang, CHEN Hong, et al. Transcriptomic analyses reveal key genes involved in pigment biosynthesis related to leaf color change of Liquidambar formosana Hance [J/OL]. Molecules, 2022, 27 (17): 5433[2024-05-09]. DOI: 10.3390/molecules27175433. [69] SUN Binmei, ZHU Zhangsheng, CAO Panrong, et al. Purple foliage coloration in tea (Camellia sinensis L. ) arises from activation of the R2R3-MYB transcription factor CsAN1 [J/OL]. Scientific Reports, 2016, 6 : 32534[2024-05-09]. DOI: 10.1038/srep32534. [70] AN Guanghui, CHEN Jiongjiong. Frequent gain- and loss-of-function mutations of the BjMYB113 gene accounted for leaf color variation in Brassica juncea [J/OL]. BMC Plant Biology, 2021, 21 (1): 301[2024-05-09]. DOI: 10.1186/s12870-021-03084-5. [71] GUAN Xiayu, WANG Wei, YE Qinghua, et al. De novo transcriptomic sequencing unraveled the molecular mechanisms of VvMybA1 underlying the alteration of Ficus lyrata leaf color [J/OL]. Acta Physiologiae Plantarum, 2019, 41 (1): 16[2024-05-09]. DOI: 10.1007/s11738-019-2809-x. [72] WANG Chongnan, JI Wenkai, LIU Yucheng, et al. The antagonistic MYB paralogs RH1 and RH2 govern anthocyanin leaf markings in Medicago truncatula [J]. The New Phytologist, 2021, 229(6): 3330−3344. [73] JENSEN R G, BASSHAM J A. Photosynthesis by isolated chloroplasts [J]. Proceedings of the National Academy of Sciences of the United States of America, 1966, 56(4): 1095−1101. [74] CHANG Qingshan, CHEN Sumei, CHEN Yu, et al. Anatomical and physiological differences and differentially expressed genes between the green and yellow leaf tissue in a variegated Chrysanthemum variety [J]. Molecular Biotechnology, 2013, 54(2): 393−411. [75] 姬语潞, 杨维, 李涵, 等. 铁皮石斛叶色突变体的叶绿体超微结构、光合色素和叶绿素荧光特性的研究[J]. 植物科学学报, 2020, 38(2): 260−268 JI Yulu, YANG Wei, LI Han, et al. Study on chloroplast ultrastructure, photosynthetic pigment and chlorophyll fluorescence characteristics of Dendrobium candidum leaf color mutant [J]. Plant Science Journal, 2020, 38(2): 260−268. [76] 许庆全, 杨凤玺, 叶庆生, 等. 墨兰‘达摩’叶艺品系光合色素质量分数、叶绿素荧光特性和叶绿体超微结构的比较[J]. 热带作物学报, 2017, 38(7): 1210−1215. XU Qingquan, YANG Fengxi, YE Qingsheng, et al. Comparison of photosynthetic pigment content, chlorophyll fluorescence characteristics and chloroplast ultrastructure of a leaf art strain of moran ‘dharma’ [J]. Chinese Journal of Tropical Crops, 2017, 38(7): 1210−1215. [77] LI Ji, WU Kunlin, LI Lin, et al. Cytological, biochemical, and transcriptomic analyses of a novel yellow leaf variation in a Paphiopedilum (Orchidaceae) SCBG COP15 [J/OL]. Genes, 2021, 13 (1): 71[2024-05-09]. DOI: 10.3390/genes13010071. [78] 杨冲, 张扬勇, 方智远, 等. 甘蓝叶色黄化突变体 YL-1 的光合生理特性及其叶绿体的超微结构[J]. 园艺学报, 2014, 41(6): 1133−1144. YANG Chong, ZHANG Yangyong, FANG Zhiyuan, et al. Photosynthetic physiological characteristics and chloroplast ultrastructure of yellow leaf mutant YL-1 in cabbage [J]. Acta Horticulturae Sinica, 2014, 41(6): 1133−1144. [79] LUO Zhongxia, ZHANG Xiongjian, CHEN Jingyi, et al. Examination and genetic analysis of a yellow-green leaf mutant a269 of sweetpotato [J]. Plant Breeding, 2020, 139(2): 381−388. [80] ZHANG Lulu, ZHANG Junkang, MAO Yunfei, et al. Physiological analysis and transcriptome sequencing of a delayed-green leaf mutant ‘Duojiao’ of ornamental crabapple (Malus sp. ) [J]. Physiology and Molecular Biology of Plants, 2022, 28(10): 1833−1848. [81] GAO Linlin, HONG Zhenghui, WANG Yinsong, et al. Chloroplast proteostasis: a story of birth, life, and death [J/OL]. Plant Communications, 2023, 4 (1): 100424[2024-05-09]. DOI: 10.1016/j.xplc.2022.100424. [82] ZAGARI N, SANDOVAL-IBAÑEZ O, SANDAL N, et al. SNOWY COTYLEDON 2 promotes chloroplast development and has a role in leaf variegation in both Lotus japonicus and Arabidopsis thaliana [J]. Molecular Plant, 2017, 10(5): 721−734. [83] HAMMANI K, TAKENAKA M, MIRANDA R, et al. A PPR protein in the PLS subfamily stabilizes the 5'-end of processed rpl16 mRNAs in maize chloroplasts [J]. Nucleic Acids Research, 2016, 44(9): 4278−4288. [84] ZOSCHKE R, WATKINS K P, MIRANDA R G, et al. The PPR-SMR protein PPR53 enhances the stability and translation of specific chloroplast RNAs in maize [J]. The Plant Journal: for Cell and Molecular Biology, 2016, 85(5): 594−606. [85] ZHANG Jian, XIAO Jianwei, LI Yuqian, et al. PDM3, a pentatricopeptide repeat-containing protein, affects chloroplast development [J]. Journal of Experimental Botany, 2017, 68(20): 5615−5627. [86] WANG Xinwei, ZHAO Lirong, MAN Yi, et al. PDM4 a pentatricopeptide repeat protein, affects chloroplast gene expression and chloroplast development in Arabidopsis thaliana [J/OL]. Frontiers in Plant Science, 2020, 11 : 1198[2024-05-09]. DOI: 10.3389/fpls.2020.01198. [87] LÜ Yang, WANG Yueying, ZHANG Qiang, et al. WAL3 encoding a PLS-type PPR protein regulates chloroplast development in rice [J/OL]. Plant Science, 2022, 323 : 111382[2024-05-09]. DOI: 10.1016/j.plantsci.2022.111382. [88] LAN Jie, LIN Qibing, ZHOU Chunlei, et al. Young Leaf White Stripe encodes a P-type PPR protein required for chloroplast development [J]. Journal of Integrative Plant Biology, 2023, 65(7): 1687−1702. [89] WANG Ying, REN Yulong, ZHOU Kunneng, et al. WHITE STRIPE LEAF4 encodes a novel P-type PPR protein required for chloroplast biogenesis during early leaf development [J/OL]. Frontiers in Plant Science, 2017, 8 : 1116[2024-05-09]. DOI: 10.3389/fpls.2017.01116. [90] LEE K, PARK S J, des FRANCS-SMALL C C, et al. The coordinated action of PPR4 and EMB2654 on each intron half mediates trans-splicing of rps12 transcripts in plant chloroplasts [J]. The Plant Journal: for Cell and Molecular Biology, 2019, 100(6): 1193−1207. [91] YAN Junjie, ZHANG Qunxia, YIN Ping. RNA editing machinery in plant organelles [J]. Science China Life Sciences, 2018, 61(2): 162−169. [92] CUI Xuean, WANG Yanwei, WU Jinxia, et al. The RNA editing factor DUA1 is crucial to chloroplast development at low temperature in rice [J]. The New Phytologist, 2019, 221(2): 834−849. [93] ZHANG Qiang, WANG Yaliang, XIE Wei, et al. OsMORF9 is necessary for chloroplast development and seedling survival in rice [J/OL]. Plant Science, 2021, 307 : 110907[2024-05-09]. DOI: 10.1016/j.plantsci.2021.110907. [94] 高贤明, 陈灵芝. 植物生活型分类系统的修订及中国暖温带森林植物生活型谱分析[J]. Acta Botanica Sinica, 1998, 40(6): 553−559. GAO Xianming, CHEN Lingzhi. The revision of plant life-form system and an analysis of the life-form spectrum of forest plants in the warm temperate zone of China [J]. Acta Botanica Sinica, 1998, 40(6): 553−559. [95] STERN D B, HANSON M R, BARKAN A. Genetics and genomics of chloroplast biogenesis: maize as a model system [J]. Trends in Plant Science, 2004, 9(6): 293−301. [96] GAO Tongmei, WEI Shuangling, CHEN Jing, et al. Cytological, genetic, and proteomic analysis of a sesame (Sesamum indicum L. ) mutant Siyl-1 with yellow-green leaf color [J]. Genes & Genomics, 2020, 42(1): 25−39. [97] TANG Yuhan, FANG Ziwen, LIU Mi, et al. Color characteristics, pigment accumulation and biosynthetic analyses of leaf color variation in herbaceous peony (Paeonia lactiflora Pall. ) [J/OL]. 3 Biotech, 2020, 10 (2): 76[2024-05-09]. DOI: 10.1007/s13205-020-2063-3. [98] HAN Hongwei, ZHOU Yuan, LIU Huifang, et al. Transcriptomics and metabolomics analysis provides insight into leaf color and photosynthesis variation of the yellow-green leaf mutant of Hami melon (Cucumis melo L. ) [J/OL]. Plants, 2023, 12 (8): 1623[2024-05-09]. DOI: 10.3390/plants12081623. [99] BRESTIC M, ZIVCAK M, KUNDERLIKOVA K, et al. High temperature specifically affects the photoprotective responses of chlorophyll b-deficient wheat mutant lines [J]. Photosynthesis Research, 2016, 130(1/3): 251−266. [100] RUAN Banpu, GAO Zhenyu, ZHAO Juan, et al. The rice YGL gene encoding an Mg2+-chelatase ChlD subunit is affected by temperature for chlorophyll biosynthesis [J]. Journal of Plant Biology, 2017, 60(4): 314−321. [101] ZHANG Haitao, LI Jinjie, YOO J H, et al. Rice Chlorina-1 and Chlorina-9 encode ChlD and ChlI subunits of Mg-chelatase, a key enzyme for chlorophyll synthesis and chloroplast development [J]. Plant Molecular Biology, 2006, 62(3): 325−337. [102] DENG Xiaojuan, ZHANG Haiqing, WANG Yue, et al. Mapped clone and functional analysis of leaf-color gene Ygl7 in a rice hybrid (Oryza sativa L. ssp. indica) [J/OL]. PLoS One, 2014, 9 (6): e99564[2024-05-09]. DOI: 10.1371/journal.pone.0099564. [103] CHEN Ping, HU Haitao, ZHANG Yu, et al. Genetic analysis and fine-mapping of a new rice mutant, white and lesion mimic leaf [J]. Plant Growth Regulation, 2018, 85(3): 425−435. [104] 王建玉, 王志鹏, 段祥坤. 甜瓜芽黄标记性状的发现与遗传分析[J]. 中国瓜菜, 2019, 32(2): 15−17. WANG Jianyu, WANG Zhipeng, DUAN Xiangkun. Discovery and genetic analysis of yellow markers in melon bud [J]. China Cucurbits and Vegetables, 2019, 32(2): 15−17. [105] WU Dianxing, SHU Qingyao, XIA Yingwu. In vitro mutagenesis induced novel thermo/photoperiod-sensitive genic male sterile indica rice with green-revertible xanthan leaf color marker [J]. Euphytica, 2002, 123: 195−202. [106] LIM S H, SOHN S H, KIM D H, et al. Use of an anthocyanin production phenotype as a visible selection marker system in transgenic tobacco plant [J]. Plant Biotechnology Reports, 2012, 6(3): 203−211. [107] LI Chuan, WANG Jingwen, HU Zhaoyong, et al. A valine residue deletion in ZmSig2A, a sigma factor, accounts for a revertible leaf-color mutation in maize [J]. The Crop Journal, 2021, 9(6): 1330−1343. [108] SIDDAPPA S, SHARMA N, SALARIA N, et al. CRISPR/Cas9-mediated editing of phytoene desaturase (PDS) gene in an important staple crop, potato [J/OL]. 3 Biotech, 2023, 13 (5): 129[2024-05-09]. DOI: 10.1007/s13205-023-03543-w. [109] NISHITANI C, HIRAI N, KOMORI S, et al. Efficient genome editing in apple using a CRISPR/Cas9 system [J/OL]. Scientific Reports, 2016, 6 : 31481[2024-05-09]. DOI: 10.1038/srep31481. [110] JEONG Y S, CHOI H, KIM J K, et al. Overexpression of OsMYBR22/OsRVE1 transcription factor simultaneously enhances chloroplast-dependent metabolites in rice grains [J]. Metabolic Engineering, 2022, 70: 89−101. [111] LIU Guofeng, HAN Zhuoxiao, FENG Lin, et al. Metabolic flux redirection and transcriptomic reprogramming in the albino tea cultivar ‘Yu-Jin-Xiang’ with an emphasis on catechin production [J/OL]. Scientific Reports, 2017, 7 : 45062[2024-05-09]. DOI: 10.1038/srep45062. [112] 金笑雨, 王艺光, 赵宏波, 等. 彩叶桂叶色变化及生理特征分析[J]. 浙江农林大学学报, 2024, 41(5): 1056−1065. JIN Xiaoyu, WANG Yiguang, ZHAO Hongbo, et al. Color change and physiological characteristics in Osmanthus fragrans colour group [J]. Journal of Zhejiang A&F University, 2024, 41(5): 1056−1065. -
-
链接本文:
https://zlxb.zafu.edu.cn/article/doi/10.11833/j.issn.2095-0756.20240397
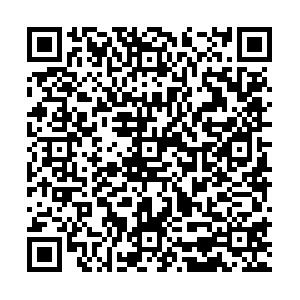
计量
- 文章访问数: 147
- HTML全文浏览量: 47
- PDF下载量: 9
- 被引次数: 0